PHARMACEUTICAL APPROACHES: ACUTE SCI |
|
Laurance Johnston, Ph.D.
Sponsor: Institute of Spinal Cord Injury, Iceland |
|
1) Methylprednisolone
2) Sygen® or GM-1 Ganglioside
3) Thyrotropin-Releasing Hormone
4) Gacyclidine
5) Neotrofin
6) Minocycline
7) Cethrin
8) Tacrolimus
9) Lipitor (Atorvastatin)
10) Erythropoietin
11) Nogo
12) Ibuprofen
13) Riluzole
14) Taxol
1)
Methylprednisolone (MP), a
synthetic glucocorticoid steroid, is often administered soon after injury
and many assume it is a post-injury standard of care, though many
scientists are now challenging that assumptio n.
This use is based on a foundation of animal studies and key clinical
studies in humans. MP minimizes post-injury neurological damage by
inhibiting, in part, lipid peroxidation, a key process that mediates
secondary damage to the
injured
cord (Click on thumbnail illustration below (From Spinal Cord Medicine
Principles and Practice, 2003, p 780))
There have been a number of MP-focused clinical
trials, including two key multi-center, randomized, double-blind,
placebo-controlled, clinical trials: National Acute Spinal Cord Injury
Study 2 (NASCIS 2) and NASCI 3. An earlier NASCIS 1 study of MP generated
no significant results.
In NASCI 2, 162 acutely injured patients
received a MP bolus of 30 mg per kilogram of body weight followed by
infusion of 5.4 mg per kilogram per hour for 23 hours. These patients were
compared to 171 patients given placebo (Bracken et al, N Engl J Med,
322, 1990). Motor and sensory function was assessed at admission and after
six weeks and six months. The investigators concluded that patients
treated with MP within eight hours of injury had improved neurological
recovery. Side effects included GI bleeding, wound infections, and delayed
healing. The study also evaluated a second drug naloxone, which did not
improve neurological function.
Before the results were published in a professional
journal, the US National Institutes of Health disseminated them through
announcements and faxes to emergency-room physicians and the news media.
Though this was done to help the acutely injured as soon as possible, it
essentially created a standard of care before other experts could
critically evaluate the results.
MP Treatment Regimen (Click on thumbnails)
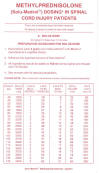 |
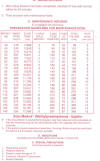 |
NASCIS 3 compared the efficacy of a
24-hour MP dose with a 48-hour dose of MP or the non-glucocorticoid
tirilazad, which was included to ascertain if it had MP’s effectiveness
without possessing MP’s steroid-related side effects (Bracken et al, JAMA
277(2), 1997). Based on the results of the previous NASCIS 2 study, all
499 acutely injured subjects were initially given 30-mg/kg dose of MP
within eight hours of injury. Then, patients were randomized to receive 1)
a 5.4-mg/kg infusion of MP for 24 hours, 2) the same dose for 48 hours, or
3) 2.5 mg/kg of tirilazad every six hours for 48 hours.
Follow-up assessments were again carried out at six
weeks and six months. The investigators concluded that if MP is initially
administered within three hours of injury, the regimen should be continued
for 24 hours; if initiated three to eight hours after injury, the regimen
should be continued for 48 hours. Patients treated with tirilazad for 48
hours had comparable improvement to the patients treated with the 24-hour
MP regimen. MP-associated side effects were greater in patients treated
for 48 hours.
Controversy: A growing number of
critics believe that MP has been promoted as a standard of care for acute
injury based on results obtained through the use of questionable
statistical procedures. Simply stated, the NASCIS 2 study showed little if
any statistically significant benefits from high-dose MP, and modest
benefits were only demonstrated in a patient subgroup when study data was
micro-analyzed in a challenged post-hoc fashion.
This controversy is not insignificant. For example, a
survey of participants at a 2001 Annual Canadian Spine Society meeting
indicated: “75% of respondents were using MP either because everyone else
does or out of fear for failing do so.”
The Canadian Spine Society and the Canadian
Neurosurgical Society commissioned an expert review of the available MP
data, which concluded that there was insufficient evidence to support the
use of MP as a treatment standard or guideline, although there is weak
clinical evidence to support its use as a treatment option. The two
societies adopted these recommendations (see website for Canadian
Association of Emergency Physicians: www.caep.ca/002.policies/002-01.guidelines/steriods-acute-spinal.htm).
Some of the published articles that challenge the use
of MP as a treatment standard include, but are not limited to, the
following:
1) Dr. Shanker Nesathurai (Massachusetts, USA) stated
that neither NASCIS 2 or 3 convincingly demonstrated MP’s benefits. “There
are concerns about the statistical analysis, randomization, and clinical
benefits… Furthermore, the benefits of this intervention may not warrant
the possible risks.” (J Trauma 45, 1998)
2) Dr. Deborah Short and colleagues (United Kingdom)
extensively reviewed the scientific literature to evaluate the evidence in
support of MP’s use (Spinal Cord, 38, 2000). They concluded: “The
evidence produced by this systematic review does not support the use of
high-dose methylpredinisolone in acute spinal cord injury to improve
neurological recovery. A deleterious effect on early mortality and
morbidity cannot be excluded by this evidence.”
3) Dr. W.P. Coleman et al (Maryland, USA) strongly
criticized both NASCIS 2 and 3 for methodological weaknesses and the lack
of data that could be critically reviewed by others (J Spinal Disord
13(3), 2000). For example, they stated: “The numbers, tables, and figures
in the published reports are scant and are inconsistently defined, making
it impossible even for professional statisticians to duplicate the
analyses, to guess the effect of changes in assumptions, or to supply the
missing parts of the picture. Nonetheless, even 9 years after NASCIS II,
the primary data have not been made public…These shortcomings have denied
physicians the chance to use confidently a drug that many were
enthusiastic about and has left them in an intolerably ambiguous position
in their therapeutic choices, in their legal exposure, and in their
ability to perform further research to help their patients.”
4) Dr. R.J. Hurlbert (Alberta, Canada) concluded:
“The use of methylprednisolone administration in the treatment of acute
SCI is not proven as a standard of care, nor can it be considered a
recommended treatment. Evidence of the drug's efficacy and impact is weak
and may only represent random events. In the strictest sense, 24-hour
administration of methylprednisolone must still be considered experimental
for use in clinical SCI. Forty-eight-hour therapy is not recommended.” (J
Neurosurg 93(1 suppl), 2000)
5) In perhaps one of the most potentially damning
criticisms, Dr. Tie Qian and colleagues (New Jersey, USA) suggested that
high-dose MP therapy may damage muscles through acute corticosteroid
myopathy (ACM) and that functional improvement attributed to MP
may merely be due to the recovery of muscle damage caused by this
extremely high dose of MP (Med Hypothesis 55, 2000). The
investigators noted that under the NASCI 3 clinical protocol, a 75-kg
acutely injured individual could receive nearly 22 gm of MP, which is the
“highest dose of steroids during a 2-day period for any clinical
condition.”
6) In a recent report, Qian and colleagues (Florida,
USA) assessed the possibility that high-dose MP could cause ACM-related
muscle damage (Spinal Cord, 43, 2005). Specifically, five acutely
injured patients who received the high-dose MP treatment regimen were
compared with three control patients, who did not meet the requirements
for MP treatment (i.e., 2 gunshot injuries and 1 arrived at hospital 8
hours after injury). ACM was assessed by muscle biopsy and
electromyography (EMG). Muscle biopsies indicated that four of the five
MP-treated patients had muscle damage consistent with ACM. EMG studies
supported these findings. In the controls, muscle biopsies were normal,
and EMG’s did not suggest myopathy. The investigators concluded that “the
improvement of neurological recovery showed in NASCIS may be only a
recording of the natural recovery of ACM, instead of any protection that
MP offers to the injured spinal cord.”
7) Studies carried
out in rats by Dr. Y. Wu et al (USA) further documented the
muscle-damaging nature of MP when used as a treatment after SCI. In
this study, rats were experimentally injured at the thoracic T9-T10
level and treated with either MP or a placebo control. Seven days after
injury, both body and muscle weight was significantly reduced in the
MP-treated rats compared to controls. The investigators concluded that
MP caused substantial muscle atrophy both above and below the the level
of injury.
8) Dr. Yasuo Ito et al (Japan) compared the
outcomes of patients who had been administered high-dose MP as part of
their acute-injury care to those who had been identically treated but
without MP. Specifically, between 2003 and 2005, all patients with
cervical injuries were treated with the standard high-dose MP protocol.
The following two years, all similarly injured patients were treated
without MP. Other than MP administration, treatment was the same in both
groups. The MP-treated group included 38 patients (30 men; 8 women) with
an average age of 55 years. The non-MP treatment group included 41
patients (33 men; 8 women) averaging 60-years old.
Neurological improvement was observed in 45% and
63% of MP-treated and non-MP-treated patients, respectively. In other
words, MP-treated patients apparently fared worse. In addition to less
improvement, the MP-treated patients had a significantly greater
incidence of pneumonia. Specifically, 50% of the MP-treated patients
developed pneumonia compared with only 27% of the non-MP-treated
patients. The investigators concluded that they “found no evidence
supporting the opinion that high-dose” MP “administration facilitates
neurological improvement in patients with spinal cord injury.” They also
added that MP “should be used under limited circumstances because of the
high incidence of pulmonary complications.”
9) In a 2011 published study, Dr. M. Aomar
Millan and colleagues (Spain) retrospectively compared the
outcomes of acutely injured patients treated with MP with patients not
treated with the drug between 1997 and 2007. Using the ASIA impairment
scale described in the appendix, neurological function was measured at
ICU admission and discharge. No statistically significant differences in
neurological recovery were noted between the groups. In addition, the
MP-treated patients had more medical complications, such as
hyperglycemia (i.e., high blood sugar) and gastrointestinal bleeding.
10) In 2012, Dr. P. Felleiter and associates
(Switzerland) published the results of a retrospective study which
compared the neurological outcomes of two groups of patients with SCI
treated at different times. In the earlier time period (2001-2003) in
which MP-treatment prevailed, 96% of 110 patients received MP. In
contrast, reflecting the concerns about MP use that emerged over time,
only 23% of of the later group (2008-2010) of 116 patients received MP.
Given the large difference in the numbers treated with MP, one would
expect much more improvement in the earlier group if MP had substantial
effectiveness. Unfortunately, this was not the case. Although the
earlier MP-emphasizing group had slightly improved neurological outcomes
compared to the later group, the difference was not statistically
significant.
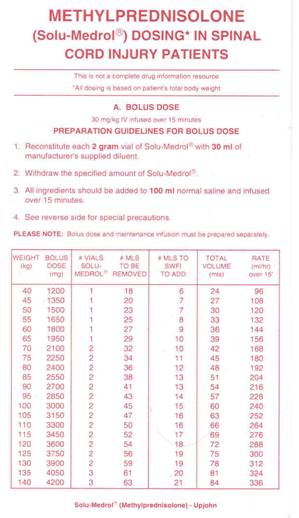
|
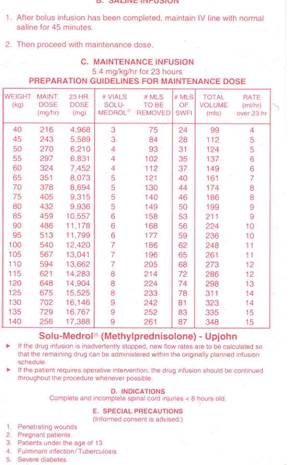
|

“There are three
kinds of lies: lies, damned lies, and statistics,” Mark Twain |
2) Sygen® or GM-1 Ganglioside:
Like MP discussed above, the degree of effectiveness of Sygen® or GM-1
ganglioside (click on thumbnail) for promoting neurological recovery after
acute SCI depends on statistical interpretation. Sygen is the trade name (Fidia
Pharmaceutical Corporation) for a naturally occurring GM-1 ganglioside, a
complex glycolipid (see figure) found in abu ndance
in central-nervous-system cell membranes.
Animal studies suggest that GM-1 exerts a
neuroprotective effect and promotes regeneration after injury. In the
acute injury phase, it prevents further cell death and injury by lessening
the consequences associated with the injury-induced over-release of
excitatory neurotransmitters, which, in turn, over-stimulates their
receptors. In the chronic injury phase, GM-1 promotes the expression of
nerve growth factors. In addition to SCI, clinical trials suggest that
GM-1 provides benefits in cases of stroke and Parkinson’s disease.
Again, like MP, GM-1’s use to treat acute SCI was the
focus of two impor tant
clinical trials. In the first, Dr. Fred Geisler et al. (Maryland,
USA) randomized patients to receive an intravenous 100-mg dose of GM-1 or
placebo within 72 hours of injury (average 48 hours) and continuing for 18
to 32 days (N Eng J Med, 324, 1991). Of the 34 subjects, 23 had
cervical injuries and 11 thoracic injuries; and 32 were men. Because all
patients were recruited at one center, the procedural variability was
inherently less than it would be in multi-center trials.
All patients were given 250-mg dose of MP upon
admission and thereafter 125 mg every six hours for 72 hours. This MP dose
was much less than that used in the NASCIS-2 study, whose results were not
known during this GM-1 study.
Follow-up assessments were carried out twice a week
for the first 4 weeks and at 2, 3, 6, and 12 months using the Frankel
scale (grade A [complete], B, C, D & E [normal motor functioning]) and the
ASIA motor score (0 = complete quadriplegia; 100 = normal motor function).
At one-year, there was statistically significant
functional improvement in the GM-1 treated patients compared to controls.
For example, in the placebo group, 13 patients stayed in the same grade, 4
improved one grade, and 1 improved two grades. In contrast, in the
GM-1-treated group, 8 stayed in the same grade, 1 improved one grade, 6
improved two grades, and 1 improved three grades. In addition, GM-1
treated patients had a statistically significant improvement in the ASIA
motor score.
In the second, six-year GM-1 trial, Giesler
and colleagues randomized 760 patients recruited from 48 North-American
SCI centers into low-dose GM-1 (331 patients), high-dose GM-1 (99
patients) and placebo (330 patients) treatment groups (Spine,
26(24S), 2001). The groups were further subdivided into six
subgroups,using 1) injury severity (grade A, B, and combined C+D) and 2)
cervical or thoracic injury.
All patients initially received the NSCIS-2 MP-dosing
regimen (i.e., the high dose; not the low dose used in the earlier GM-1
study). GM-1 treatment was not initiated until MP treatment was completed,
on average 55 hours after injury. The low-dose GM-1 regimen consisted of
an initial 300-mg loading dose followed by 56 days (i.e., 8 weeks) of a
100-mg/day; the high-dose doubled these amounts. Enrollment into the
high-dose treatment group was terminated early on.
Follow-up assessments were performed at 1, 2, 4, 6,
and 12 months. The study’s pre-defined primary measurement of GM-1
efficacy was the proportion of patients at 6 months with “marked
recovery,” defined using various SCI-assessment scales. A secondary
efficacy measure included the time course of marked recovery and other
established measures of spinal cord function.
Study results were mixed and depended on the time of
assessment. For example, although the GM-1-treated group did not have a
statistically significant greater number of patients with “marked
recovery” at 6 months (i.e., study’s primary efficacy measure), it had
statistically significant greater recovery at the end of the two-month
dosing period, suggesting that GM-1 accelerates recovery that is obtained.
The drug appeared to enhance ASIA motor, light touch, and pinprick scores,
as well as bowel function, sacral sensation, and voluntary anal
contraction. Finally, GM-1 appeared to exert greater effects on
individuals with incomplete injuries.
Although statistical significance was not shown for
the pre-defined, primary endpoint, the investigators, nevertheless,
believed that GM-1 is “active in SCI, somehow: in some respect, using some
regimen, and in some group of patients.” The possibility was suggested
that MP and GFM-1 may have antagonistic actions, and, as such, the much
higher, NSCIS-2-mandated MP dose administered in the second GM-1 trial may
have diminished GM-1’s beneficial effects.

3) Thyrotropin-Releasing Hormone
(TRH) is a three amino-acid peptide (glutamic acid-histidine-proline)
produced in the brain’s hypothalamus. In spite of its molecular
simplicity, TRH influences diverse biological function through affecting
the secretion of a variety of hormones, including thyroid hormone,
prolactin, growth hormone, vasopressin, and insulin; and the
neurotransmitters noradrenaline and adrenaline.
Experimental studies in a animal models indicate that
TRH improves long-term motor recovery after acute, apparently by
minimizing some of the biochemical and physiological processes that
mediate secondary injury.
In 1995, Dr. Lawrence Pitt and colleagues
(California, USA) reported the results of treating 20 acutely injured
patients with TRH recruited over a two-year period from 1986–1988 (J
Neurotrauma 12(3), 1995). The patients were subdivided into four
groups: 1) complete and incomplete injuries and 2) in a double-blind
fashion, TRH- or placebo-treated groups. The treatment groups were dosed
with 0.2 mg/kg intravenous bolus of TRH followed by an hourly infusion of
the same dose for six hours.
Patients were examined at various follow-up periods
up using 1) motor and sensory scales in which 0 corresponded to no
movement and 5 normal power, and 1 corresponded to no sensation and 3
normal sensation; and 2) a 1-10 Sunnybrook scale in which 1 represented
complete motor and sensory loss, and 10 represented normal motor and
sensory function.
At the four-months before patient attrition started
compromising the study, the TRH-treated group with incomplete
injuries demonstrated improved functional recovering using the
aforementioned scales. However, no improvement was noted in the
TRH-treated group with complete injuries. The investigators
emphasize that results must be interpreted with caution due to the small
sample size.
Unfortunately, in spite of the pilot-study’s positive
results and many promising animal studies, no further work in humans with
SCI appears to have been carried out. TRH efforts are now focusing on
traumatic brain injury.

4) Gacyclidine: Dr.
Alain Privat and colleagues (Montpellier, France) have studied the
effectiveness gacyclidine in minimizing neurological damage after acute
SCI. Basically, after injury, cells lyse, releasing excitatory amino
acids, such as glutamate, which soon reach toxic concentrations. Through
interactions with receptors on neighboring cells, excessive glutamate will
initiate a neurotoxic biochemical cascade. Animal studies suggest that
antagonists that block these receptors will exert a neuroprotective
influence by inhibiting this cascade. Gacyclidine is one of these
antagonists.
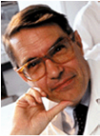
Privat’s study recruited over 200 patients, a
relatively large clinical trial for SCI. Most subjects were treated within three hours of injury and
once again in the following four hours. Although analysis of the study
results indicated no statistically significant difference between the gacyclidine and placebo-treated groups, data suggested that subjects who
1)received the highest drug dose functionally improved, and 2) sustained
cervical injuries had the most improvement.

5) Neotrofin®:
NeoTherapeutics (Irvine, California) sponsored clinical trials examining
the effect of Neotrofin (also called AIT-082 or leteprinim) on several
neurological disorders, including Alzheimer’s disease, Parkinson’s
disease, and SCI. Neotrofin is a purine analog that can be taken orally,
and due to its relatively small size, able to cross the blood-brain
barrier, a prerequisite for any drug that targets central nervous system
(CNS) disorders.
Animal studies indicate Neotrofin reduces
neurological damage and improves walking after acute injury in rats (Middlemiss,
et al. Soc Neurosci Abstr, 25, 1999). Evidence suggests that
Neotrofin stimulates the production of neurotrophic factors, such as nerve
growth factor and protects neurons from glutamate-induced cell death (see
previous section). Studies in mice indicate that the drug also stimulates
the proliferation of CNS stem cells, which given the increasing number of
SCI-related stem-cell programs may have important implications.
In 2001, NeoTherapeutics started the recruitment of
an intended 30-40 patients with complete SCI one to three weeks after
injury (i.e., sub-acute injury phase) at four SCI centers: Thomas
Jefferson University (Pennsylvania), Craig Rehabilitation Center
(Colorado), Rancho Los Amigo (California), and Gaylord Hospital
(Connecticut). Patients orally consumed a twice daily 250-mg dose of
Neotrofin for 12 weeks.
Unfortunately, few, if any, study results have been
reported. After Neotrofin failed to demonstrate a statistically
significant effect in late-stage clinical trials for Alzheimer’s disease,
the nearly broke NeoTherapeutics reorganized into Spectrum Pharmaceuticals
with an emphasis on cancer drugs.

6)
Minocycline: A broad-spectrum antibiotic in the tetracycline
group, minocycline is often used to treat acne and rosacea. Compared to
other tetracyclines, minocycline is eliminated more slowly from the body,
and, hence, exerts a longer physiological effect.
Studies in animal models of acute SCI indicate that
minocycline minimizes the secondary neurological damage that occurs soon
after injury. For example, University of Calgary researchers have shown
that acutely injured, minocycline-treated mice recover more hind-limb
function and strength compared to untreated mice. In addition, minocycline
reduced the size of the injury-site lesion and promoted the survival of
axons through the injury site (Wells JE, et al, Brain, 126(7),
2003). Harvard University (Teng YD et al, Proc Natl Acad Sci,
101(9), 2004) and South Korean investigators (Lee SM et al, J
Neurotrauma, 20(10), 2003) have also demonstrated similar
neuroprotective effects in rats.
Minocycline mediates its neuroprotective effects
through a number of mechanisms, including minimizing the destructive,
post-injury immune response and the release of cytochrome c. Although
cytochrome c is a key cell-respiratory protein essential for cell life,
when it is released, it initiates a metabolic cascade triggering cell
death.
Based on the results of these animal studies, Dr.
R. John Hurlbert, Dr. Steven Casha, and colleagues (Canada) have
initiated a randomized, double-blind, placebo-controlled, phase-1 and -2
studies evaluating minocycline administered within 12 hours of injury.
Sixty subjects with injuries at or above the thoracic T11 level are to
be cumulatively recruited. The minocycline will be intravenously
administered in twice-daily doses for seven days. Control subjects would
receive a saline infusion. The patients will be followed for a two years
using a variety of assessments, including ASIA motor and sensory scores,
the Quality-of-Life Assessment, and the Functional Independence Measure.
Phase II results were reported in 2012. The study
included 27 minocycline-treated patients (22 men and 5 females) and 25
placebo-treated controls. Average age of the minocycline patients was
41, and their injury cause was motor vehicle accidents (14), sport
injuries (6), work accidents (5), and falls (2). Sixteen and 11 had
cervical and thoracic injuries, respectively. Among other measurements,
neurological function was periodically assessed for up to a year using
ASIA motor scores. After three months of evaluations, minocycline-treated
patients averaged six points more of motor recovery than controls. The
effects of minocycline treatment seemed more robust for those with
cervical injuries. Specifically, minocycline-treated patients with
cervical injuries averaged 14 points more motor recovery than controls,
a difference that approached statistical significance. In contrast,
those with thoracic injuries accrued minimal benefit. The investigators
concluded: “The minocycline regimen established in this study proved
feasible, safe, and was associated with a tendency towards improvement
across several outcome measures.”
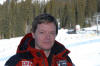
Dr. R. John Hurlbert |
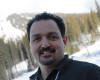
Dr. Steven Casha |

7) Cethrin®
Based on a foundation of research by
Dr. Lisa McKerracher et al (Canada), BioAxone/Alseres Pharmaceuticals has
initiated clinical trials evaluating Cethrin in patients with acute SCI.
Animal studies indicate that SCI stimulates the
production of Rho, a molecule that inhibits axonal growth and
regeneration, and initiates a physiological cascade that results in the
death of nearby neuronal cells (a process called apoptosis). Cethrin
apparently blocks these adverse effects, restoring regenerative
potential and preventing cell death.
The initial clinical trial was designed to test
Cethrin’s safety and pharmacokinetics at various dosing regimens, and
did not include the control subjects necessary to determine overall
effectiveness. The drug was administered an average of 53 hours after
injury at the time of spinal-stabilization/decompression surgery, a
procedure often done relatively soon after injury. As the surgery is
being completed, Cethrin was applied on the membrane covering the spinal
cord using a sealant to keep it in place. The drug penetrates through
the membrane to the underlying neuronal tissue.
As reported in 2011, 48 subjects were recruited at
nine clinical sites in the United States and Canada. Thirty-two had
thoracic injuries (mean age 34) and 16 had cervical injuries (mean age
41). All subjects (40 men, 8 women) had ASIA-A complete injuries (most
complete on a scale ranging from ASIA-A to ASIA-E representing total
recovery). In addition to assessing recovery by this impairment scale,
motor-function gains were periodically followed for a year. Of the 48
subjects initially enrolled, 35 completed the study.
Overall, the results suggested that patients with
cervical injuries accrued greater benefit from Cethrin treatment.
Specifically, although only 6.3% of subjects with thoracic injuries
improved two levels to ASIA-C functioning (i.e., some sensory and motor
recovery) after one year, 31% of those with cervical injuries did so. In
one of the dosing regimens, improvements were especially impressive,
with 66% improving to ASIA-C. Two subjects with cervical injuries and
one with a thoracic injury improved three levels to ASIA-D. For the
sake of comparison, historical data suggests that less than 10% of
individuals with cervical injuries would spontaneously improve two or
more grades.
Using a scale ranging from 0 to 100, motor scores
on average improved 18.6 points for subjects with cervical injuries
compared with historical data suggesting only a 10-point improvement is
likely after a year. Again, one dosing regimen appeared especially
effective with a 27-point improvement. In contrast, little motor
improvement was noted in subjects with thoracic injuries.
Alseres Pharmaceuticals has indicated their
intention to initiate a much larger study, which will recruit 200
subjects with cervical C5-7, ASIA-A complete injuries.

8)
Tacrolimus & Minocycline:
Although available details are scant, investigators at Riyadh Armed Forces
Hospital, Saudi Arabia have initiated a clinical trial assessing the
effectiveness of tacrolimus and minocycline in reducing neurological
damage after acute SCI. Minocycline is discussed above. Tacrolimus (also
called FK506) is an antibiotic originally obtained from soil bacteria. It
is primarily used to 1) suppress the immune system to minimize rejection
of transplanted organs and 2) topically to treat eczema. Animal studies
suggest that it exerts a neuroprotective effect that may be greater than
the currently widely used methylprednisolone (Exp
Neurol 1998; 154(2);
Exp Neurol
1999; 158(2); Exp Neurol
2002; 177(1); & J Neurosci Res
2005; 81(6)). Tacrolimus inhibits calcineurin, an enzyme found in neurons
and lymphocytes (white blood cells). This inhibition apparently prevents
lymphocyte activation, in turn attenuating the destructive, post-injury
immune process. 
9) Lipitor
(Atorvastatin): A cholesterol-lowering medicine belonging to the
statin-drug group, Lipitor (trade name for atorvastatin)
is one of society’s most widely used and profitable drugs. By inhibiting
the liver’s enzymatic production of a cholesterol precursor, it lowers
cholesterol levels. Animal and clinical studies suggest that Lipitor or
related statins exert a neuroprotective and anti-inflammatory influence
for various neurological disorders, including MS, Alzheimer’s disease,
stroke, and SCI.
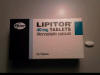
Drs. Avtar and Inderjit Singh and colleagues (South
Carolina, USA) have carried out several studies indicating that Lipitor
minimizes neurological damage in rats with an experimental contusion
injury (i.e., comparable to the sort of injury observed in most
humans). In the first study, rats treated before and after injury
with Lipitor recovered more hind-limb function than control animals (J
Neurosci Res. 79(3), 2005).
A more recent study showed that this
neuroprotective effect was also observed when the rats were given
Lipitor only after injury, a finding, of course, needed if the drug is
to have any real-world applicability.
Specifically, rats were given oral doses of Lipitor two, four, or six
hours after injury followed thereafter by a once daily dose. Compared to
controls, Lipitor-treated rats regained considerable recovery in
hind-limb function, with the earlier treated rats regaining the most.
Apparently, Lipitor helped preserve the
blood-spinal-cord barrier after injury, which, in turn, limited the
infusion into the injury site of inflammatory molecules that cause
function-compromising secondary damage. Overall, there was more tissue
sparing in Lipitor-treated rats, including less 1) degeneration of
neuronal axons, 2) degradation of the conduction-promoting,
axon-insulating myelin sheath, 3) scar-forming gliosis (the production
of a dense complex of neuronal support cells called glia in the injury
area), and 4) neuronal cell death
through apoptosis (below).
Lipitor also suppresses the injury-induced
expression of Rho (see discussion above), a molecule that inhibits
axonal growth and regeneration, and initiates a physiological cascade
that results in the death of nearby neuronal cells.
Dr. M.A. Dery and colleagues (Canada) have
studied Lipitor’s influence on neuronal cell apoptosis and recovery of
locomotion in rats with SCI. As discussed elsewhere, apoptosis is a form
of secondary cell death in which a programmed sequence of events leads
to cell elimination. In this study, rats were injected with either a
Lipitor or a saline solution intraperitoneally (i.e., into the body
cavity) two hours after injury experimentally produced by contusion, a
type of injury similar to that frequently observed in humans with SCI.
Four hours post-injury, Lipitor-treated rats had 20% fewer neuronal
cells dying through apoptosis compared to controls, and apparently as a
consequence, four weeks after injury, they demonstrated greater recovery
of locomotion.

10)
Erythropoietin (EPO): EPO (Epogen®) is a
glycoprotein growth hormone produced by the kidney that stimulates the
bone-marrow production of red blood cells. In a feedback fashion,
decreases in blood-oxygen levels trigger EPO synthesis, which, in turn,
results in the creation of more oxygen-carrying red blood cells. EPO has
been primarily used in the treatment of kidney disease, in which EPO
production has been compromised, and cancer to ameliorate the side
effects of chemotherapy- or radiation-induced anemia. Because of EPO’s
ability to promote oxygenation, endurance athletes have used the drug as
a blood-doping agent to obtain a competitive advantage.
More recently, it has been shown that EPO is also
created by the CNS and exerts a neuroprotective influence for a variety
of neurological disorders, including stroke, head injury, and SCI. In
the case of SCI in animal models, a number of interacting physiological
mechanisms may mediate EPO-influenced neuroprotection: Specifically, EPO
 | blocks injury-related cell death called
apoptosis; |
 | prevents injury-related hypoxia in which
limited oxygen reaches the spinal cord; |
 | inhibits the damage caused by excitotoxins,
amino acids which, when elevated by injury, damage neighboring
neuronal cells; |
 | reduces injury-site inflammation; |
 | restores post-injury vascular integrity,
enhancing blood flow and, in turn, tissue oxygenation; |
 | enhances neuronal regeneration through the
stimulation of stem cells to produce new neurons and support cells. |
Given EPO’s well-documented neuroprotective effect
in injured animals, Italian investigators have initiated a multicenter
clinical trial comparing the effectiveness of EPO with
methylprednisolone (MP, discussed previously) for treating acute SCI.
The study intends to recruit 100 subjects at Italian SCI centers within
eight hours of injury and randomize them to receive either MP or EPO.
All subjects must have more complete ASIA A-B injuries (i.e., the most
neurologically complete injuries; see appendix). Relative improvement
will be periodically measured over three months using this
ASIA-impairment scale, as well as assessments evaluating neuronal
conduction (i.e., electrophysiology), spasticity, pain, and functional
autonomy.

11) Anti-Nogo:
Since the 1980’s, Dr. Martin Schwab and colleagues
(Switzerland) have been investigating factors that inhibit neuronal
regeneration after injury and, in turn, the blocking of these factors as
a means to promote functional recovery.
His
research was based on the findings by others, which showed that usually
regeneration-reluctant, spinal-cord neurons are able to grow when placed
in the peripheral-nervous-system (PNS - nerves outside of the brain and
spinal cord). These findings suggested that a CNS environmental factor
inhibited neuronal regeneration which did not exist in the PNS. Schwab
demonstrated the factor was associated with CNS myelin, the insulating
material surrounding neurons. CNS and PNS myelin are dissimilar,
generated by different cells called oligodendrocytes and Schwann cells.
The CNS oligodendrocyte-derived myelin possesses a protein dubbed Nogo
that repels the growth cones of neuronal axons attempting to regenerate.
To negate this inhibition, Schwab’s team developed an antibody that
complexes with Nogo, hence, neutralizing or blocking Nogo.
As a crude analogy, visualize the Nogo-growth
inhibitors as burrs sticking to your clothing unless they are so filled
with lint that they fall away. Essentially, the anti-Nogo antibodies
represent the molecular lint that preferentially sticks to the Nogo
burrs, keeping them from attaching to struggling growth cones. These
regenerating cones can now move forward in the spinal cord with less
inhibitory drag.
In several SCI animal models, including rats and
primates, Schwab and colleagues have shown that anti-Nogo antibodies
promote functional recovery. This recovery is apparently due to not only
the regeneration of injury-affected neurons but the stimulation of
sprouting in neurons bypassing the injury site. Based on these
experiments, the Swiss company Novartis in collaboration with Schwab has
initiated preliminary clinical trials in humans injured within 10 days
of treatment.

12)
Ibuprofen: Even though
ibuprofen’s SCI-treatment potential has only been explored in animal
models (like Lipitor discussed above), this drug is included in this
discussion because its widespread consumption makes it much easier
candidate to be considered for SCI applications. Marketed under many
brand names (e.g., Advil, Motrin, etc), ibuprofen is categorized as a
non-steroidal anti-inflammatory drug (NSAID – like aspirin). The drug
works by inhibiting the production of prostaglandins, which mediate
inflammation.
As is the case with both Cethrin and Lipitor
summarized before, ibuprofen blocks the injury-triggered production of
Rho. Again, Rho is a protein that inhibits axonal growth and
regeneration, and initiates a physiological cascade that ultimately
results in the death of nearby neuronal cells (a process called
apoptosis).
Dr. Shuxin Li and colleagues (USA) have
shown that by blocking Rho, ibuprofen stimulates the growth of not only
neurons grown in culture but within the injured spinal cord (31).
Specifically, subcutaneous injection (via mini-pump) of ibuprofen into
rats with experimental SCI stimulated considerable axonal sprouting.
Compared to untreated control animals, ibuprofen-treated rats regained
additional walking ability. The investigators concluded that “ibuprofen
promotes a remarkable locomotor functional recovery, even when
delivered 1 week after trauma. The axon growth-promoting effect and the
common use of ibuprofen in patients raise the high possibility that” it
may be an effective SCI treatment.
Dr Stephen Strittmatter et al (USA)
confirmed and extended these findings. Specifically, pumps implanted
under the skin in rats with an experimental contusion injury delivered
ibuprofen for 28 days starting three days after injury. When adjusted
for weight, the ibuprofen dosing in these rats was comparable to that
consumed by many humans on an ongoing basis. The investigators showed
that ibuprofen 1) not only blocks the Rho-regeneration inhibitor
discussed above, but also a myelin-associated glycoprotein that hinders
neuronal outgrowth; 2) stimulates the sprouting of some, but not all,
types of axons; and 3) protects and spares spinal-cord tissue at the
injury site. Compared to controls, more ibuprofen-treated rats were able
to walk; twice as many were able to support their weight with their hind
limbs. This function-restoring effect persisted well after ibuprofen
treatment had been discontinued, indicating that it was due to a
persistent change in neuronal anatomy or function and not just a
transient drug effect. 
13)
Riluzole: Dr. Robert Grossman (USA) and colleagues at several
North American SCI centers have initiated a preliminary clinical trial
evaluating the safety and pharmacokinetics (i.e., how a drug is
metabolized by the body) of treating 36 patients with SCI with riluzole.
Patients with C4-T12 level injuries will be administered 50 milligrams
of the drug twice a day for two weeks starting within 12 hours of
injury. In addition to the safety and pharmacokinetic focus, a
preliminary assessment of functional outcomes will be undertaken for the
sake of planning a more comprehensive, phase-II efficacy study.
Riluzole exerts neuroprotection through several
mechanisms, including 1) limiting the post-injury influx of damaging
sodium ions into neurons by blocking the channels through the membrane
by which the ions enter or 2) inhibiting the injury-triggered release of
certain excitatory amino-acid neurotransmitters, which at
excessive levels are neurotoxic.
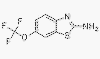
Numerous clinical trials have targeted riluzole’s
ability to treat a wide-range variety of neurological disorders,
including amyotrophic lateral sclerosis (ALS), multiple sclerosis,
schizophrenia, Parkinson’s Disease, Tourette syndrome, depression, etc.
The SCI-focused clinical trial is based on a
foundation of promising animal studies, including the following:
1) Dr. J.M. Stutzmann et al.
(France) evaluated the effects of 10 days of riluzole treatment
in rats with thoracic injuries generated by spinal cord compression.
Compared to controls, riluzole-treated rats recovered more function,
demonstrated improved nerve conduction through the injury site, and had
less tissue damage at the injury site.
2) Dr. Xiaojun Mu and associates (USA)
treated rats with a T10-level contusion injury with riluzole,
methylprednisolone (MP- discussed above), or a combination of the two.
Riluzole was intraperitoneally administered (i.e., into the body cavity)
two and four hours after injury and thereafter daily for a week. MP was
intravenously administered just two and four hours after injury.
Neuroprotective potential was assessed by evaluating 1) recovery of
hind-limb function, 2) tissue sparing at the injury site, and 3) the
amount of myelinated axons, an indication of functional neurons. Given
study constraints, only the combined MP/riluzole treatment was shown to
improve hind-limb function and spare injury-site tissue.
3) Drs. Gwen Schwartz and Michael Fehlings
(Canada) treated injured rats with intraperitoneal doses of riluzole and
several other similar acting drugs. The drugs were administered 15
minutes after an experimental injury produced by clipping the exposed
spinal cord at the C7-T1 region.
After
hindlimb function was evaluated weekly for six weeks, the animals were
sacrificed and the degree of tissue sparing anatomically examined.
Functional recovery and tissue sparing was significantly enhanced in
riluzole-treated animals. The investigators concluded that “Riluzole
should be considered an important therapeutic candidate for this form of
CNS trauma.”
4) Dr. Ozkan Ates and colleagues (Turkey)
treated injured rats with riluzole or other neuroprotective agents. A
single drug dose was intraperitoneally administered immediately after an
experimental contusion injury was produced at the T7-10 level. Various
scales were used weekly for six weeks to assess motor-function recovery.
In addition, anatomical and biochemical assessments of injury site
damage were carried out. Compared to saline-dosed controls,
riluzole-treated rats 1) recovered more motor function, 2) had smaller
injury-site lesions, and 3) had less lipid peroxidation, a biochemical
process which mediates secondary neurological damage to the injured
cord.
5) Dr. Patrick Kitzman (USA) examined
whether riluzole can reduce SCI-generated tail spasticity in rats. The
spinal cords of the rats were transected at the sacral S2 level, a type
of injury which consistently produces spasticity in tail muscles. The
animals were treated for three days with either a saline placebo
solution or two different riluzole dosages. Depending upon the dose,
riluzole-treated animals had less tail spasticity. 
14) Taxol:
Originally isolated from the bark of the Pacific Yew tree,
Taxol is a drug used to treat a variety of cancers, including lung,
ovarian, breast, and head and neck cancers. Animal research indicates it
may be also beneficial for SCI. Because of its extensive use as a cancer
treatment, much is already known about Taxol’s interactions with the
human body. In theory, such understandings should accelerate its
consideration as a SCI treatment.
Microtubules: Through stabilizing
key structural components within the cell called microtubules, Taxol
interferes with the division of cancer cells and, hence, slows tumor
growth. Microtubules are, so to speak, the protein “girders” that help
create cell infrastructure and, depending upon how these girders are
assembled, fate. Basically, Taxol stabilizes this infrastructure by
strengthening girder connections with additional rivets in the form of
molecular bonds. In the case of cancer, these rivets prevent the cells
from assuming the more malleable structure required for cell division
and, in turn, tumor growth.
As
discussed below, with SCI, Taxol stabilizes microtubule structure after
injury in a way that increases the regenerative potential of damaged
neuronal axons.Growth Cones vs.
Retraction Bulbs: Considerable animal research evaluating Taxol’s
regenerative influence on neurons has been carried out by Dr. Frank
Bradke and colleagues (Germany and USA). Much of their research has
focused on a damaged neuron’s propensity to develop either a growth
cone or retraction bulb after injury. Generally, regenerating
neurons, especially those in the regeneratively inclined peripheral
nervous system (i.e., outside the brain and spinal cord), have a growth
cone at their axonal tips. This cone contains an abundance of the
physiological machinery and substrates necessary for axonal elongation.
In contrast, damaged central-nervous-system neurons usually form
non-regenerating swellings called retraction bulbs at the tip of their
axonal stumps - essentially the non-growing equivalent of growth cones.
A key difference between growth cones and
retraction bulbs involves the degree of microtubule assembly. When
assembled in parallel bundles, microtubules are the backbone of axonal
shafts with growth cones. They lay the tracks for 1) bringing in needed
molecules, cellular structures, and energy supplies to the rapidly
advancing growth cone and 2) helping to push the axon forward. In
contrast, retraction-bulb microtubules are disorganized; rather than a
backbone, it’s more like a “collection of unconnected vertebra” that
can’t support growth.
If microtubule structure is experimentally
destabilized in a growth cone, it becomes more like a refraction bulb,
and, as a consequence, axonal growth stops. On the other hand, Taxol-induced
microtubule stabilization reduces retraction-bulb formation after CNS
injury. After Taxol treatment, the axonal endings now resemble growth
cones and are better able to push through the inhibitory, environmental
gauntlet characteristic of the injury-site scar.
Scar Reduction: In an article published in
the prestigious Science Magazine, Bradke et al provided evidence
that in addition to microtubule stabilization, Taxol reduces scarring
after SCI.
Specifically,
the investigators demonstrated that Taxol treatment reduces the amount
of regeneration-inhibiting substances that accumulate at the injury-site
scar. As a result, the environmental gauntlet that the axon must pass
through to reach its target has been diminished. Specifically, the
number of axons making it through increased five fold after Taxol
treatment, and, as a result, treated animals with SCI regained 3.4-times
more ambulatory ability than controls.
TOP
|
|
|
|
|
|
|
|
|
|
|