1) Creatine: SCI Strength
Enhancer
2) Vitamin D & SCI Bone
Density
3) Ginkgo Biloba's
Neuroprotective Effect
4) Fasting
5) Buyang Huanwu Decoction
6) Quercetin
7) Vitamin E
8) Chinese Skullcap
9)
Acetyl-L-Carnitine
10) Herbal Formulation JSK

CREATINE: SCI STRENGTH ENHANCER:
Creatine is a common nutritional supplement used by
athletes to build-up muscles and strength. Several scientific studies
suggest that creatine can also en
hance
strength in individuals with physical disability, including SCI.
Furthermore, animal studies indicate that creatine exerts a
neuroprotective effect after injury.
Our bodies contain more than 100 grams of creatine,
mostly in our muscles, heart, brain, and testes. Physical activity
stimulates primarily the liver to produce about two grams of creatine
daily from three key amino acids: glycine, arginine, and methionine. The
creatine is then sent through the blood and transported into muscle
cells.
Creatine can also be provided by diet, especially
one rich in meat and fish. Vegetarian diets, however, often lack not
only creatine, but also the methionine precursor needed for internal
production. For comparison’s sake, a pound of meat contains about
40-times more creatine than a pound of milk.
Creatine-Generated Energy: Most muscle
creatine is converted into the energetically powerful creatine-phosphate.
The high-energy molecular bond connecting the creatine to the phosphate
group is an energy source that can quickly fuel muscle activity. This
fueling, however, is mediated through the creation of yet another
powerhouse molecule called adenosine triphosphate (ATP).
ATP is extremely
important because it is the body’s energy currency, expended to drive most
biochemical processes. Like creatine-phosphate, ATP’s terminal phosphate
group is connected by a high-energy bond that when severed provides energy
needed for muscle contraction.
Under more constant or endurance working conditions,
the body obtains ATP by metabolizing carbohydrates and fats, a relatively
slow process that cannot generate immediately needed ATP energy.
When energy bursts are required, the body uses
instead creatine-phosphate. Specifically, the phosphate group on this
molecule is transferred to replenish spent ATP, transforming it into its
energetically powerful form. During rest periods, creatine-phosphate is
then replenished by the ATP generated by the slower metabolic processes.
If intracellular creatine-phosphate levels can be
increased, for example, through supplementation, it will take longer
before the short-term energy source is depleted and a switchover to slower
carbohydrate or fat metabolism is needed.
Strength & Muscles: Creatine
supplementation is most useful for physical activities that require
intense bursts of energy - e.g., a bench press, a sprint, or games
requiring energy bursts. It is less useful for endurance events, except
when such events are enhanced by building-up muscle strength through
creatine-stimulated weightlifting.
Creatine can build muscle mass by several mechanisms.
For example, because weightlifting is exactly the sort of short-term,
intense physical activity fostered by creatine, more repetitions and
harder workouts can be achieved, building up muscle. In addition, however,
creatine increases water uptake into the muscle, a process called cell
volumizing that bulks up the muscles in a fashion that may not add much
real strength.
Physical Disability & SCI: Studies
suggest that creatine can enhance strength compromised by physical
disability:
First, Dr. P. Jacobs and colleagues at the Miami
Project have shown that creatine promotes upper-extremity work capacity
in quadriplegics ((Arch Phys Med Rehabil 83, 2002). In this study,
16 male quadriplegics with complete cervical C5-7 injuries were randomly
assigned to receive either 20 grams/day of creatine or placebo
maltodextrin (a common food ingredient) for seven days. Treatment was then
discontinued for a three-week washout period, after which the treatment
groups were reversed for another seven days - i.e., the initial placebo
group now received creatine, and the initial creatine group now was given
maltodextrin.
Work capacity was assessed before and after each
dosing period using arm ergometry, a common SCI-rehabilitation exercise.
Specifically, subjects faced a series of two-minute, increasing-intensity
work stages with one-minute, intervening recovery periods.
After creatine supplementation, improvements were
noted in various respiratory measurements, including oxygen uptake, carbon
dioxide production, tidal volume (amount of air that enters the lungs),
and breathing rate. For example, 14 of the 16 subjects demonstrated
increased oxygen uptake, averaging 19 %. Improvements were also noted in
peak power output and increased time to fatigue.
Second, Dr. K. Adams et al (Dallas, Texas) carried
out a creatine-loading study in 10 subjects with SCI (Arch Phys Med
Rehabil 81, 2000). The subjects had their peak-power production tested
on an upper extremity exercise machine before and after creatine
supplementation. Most improved their peak-power production, with
quadriplegics and paraplegics averaging 21and 13% improvement,
respectively.
Third, Drs. Stephen Burns, R. Kendall and
colleagues (USA) examined the effects of creatine supplementation on
muscle strength in eight subjects with quadriplegia. Average age was 48,
seven were men, and seven had C6-level injuries. In a double-blind
crossover study (a study design in which subjects unknowingly become
controls and vice versa during the study), subjects were randomized to
receive either creatine supplementation or a placebo Wrist and grasping
strength were evaluated before and after supplementation. Unlike the
preceding studies, results suggested that no additional strength accrued
from creatine supplementation.
Finally, Drs. M. Tarnopolsky and J. Martin (Hamilton,
Ontario) have shown that creatine can increase handgrip, knee-extension,
and ankle strength in individuals with various forms of neuromuscular
disease (Neurology 52, 1999).
Neuroprotection: Animal studies
indicate that creatine exerts a neuroprotective effect in traumatic brain
and spinal cord injury. For example, Dr. O. Hausmann et al (Zurich,
Switzerland) demonstrated that four-weeks of creatine supplementation
before experimental spinal cord injury reduced glial scar formation and
enhanced functional recovery in rats. In
another example, Dr. A. Rabchevsky and colleagues (Lexington, Kentucky)
showed that creatine supplementation spared spinal cord gray matter in
injured rats (gray matter contains neuronal cell bodies and dendrites and
glial cells; white matter consists mainly of axons).

VITAMIN D &
BONE DENSITY: As summarized in two key articles, research
carried out by Dr. William Bauman and colleagues, Bronx VA Medical Center
indicates that individuals with SCI are often vitamin-D deficient (Metabolism
44(12), 1995; & J Spinal Cord Med 28, 2005).
Like astronauts who lose bone density from the lack
of weight-bearing activities, paralysis causes osteoporosis. As much as
50% of lower-extremity bone mass is lost during the first several years
after injury, people with complete injuries losing the most. Hence, a
deficiency in bone-enhancing vitamin D further aggravates an already
serious SCI problem, in turn increasing fracture risk.
Bauman believes SCI predisposes one to vitamin-D
deficiency for several reasons. For example, he speculates that due to
limited mobility, someone with SCI may not get as much vitamin-D-producing
sunlight as the general population. Supporting this idea, other scientists
have demonstrated that pressure-sore-afflicted patients with SCI, who have
access to the least sunlight, have the greatest vitamin-D deficiency.
Bauman also suggests that a lack maybe be caused when
health-care professionals recommend reduced consumption of
vitamin-D-fortified dairy products under the mistaken belief that the
calcium in such foods will aggravate kidney problems. And, he believes
that many SCI-associated medicines reduce the body’s vitamin-D stores.
In his 1995 study, Bauman compared vitamin-D levels
in control subjects and in 100 veterans with SCI who averaged 20 years
post-injury. Subjects with SCI were twice as likely to have vitamin-D
levels less than that considered normal.
In his 2005 study, Bauman examined the effectiveness
of several dosing regimens in elevating vitamin-D levels in people with
chronic SCI. In one regimen, 40 subjects consumed 800 IU of vitamin-D per
day for 12 months. Their mean age was 43; injury duration averaged 12
years; and 17 and 23 had quadriplegia and paraplegia, respectively. Before
supplementation, 33 had below-normal vitamin-D levels; in
contrast, after
12 months of supplementation, only 9 remained deficient.
Although average serum vitamin-D levels doubled in
subjects, Bauman believes that even greater supplementation is needed to
obtain nutrient serum levels needed for promoting optimal bone health in
SCI.
Dr. Christina Oleson and colleagues (USA)
assessed vitamin-D levels in individuals with SCI from the Birmingham,
Alabama area. Because Birmingham is in the southern USA, solar exposure
in the area includes more of the sun’s vitamin-D-producing ultraviolet
UV-B rays compared to most other parts of the country. Hence, any
vitamin-D deficiencies observed in this location would suggest that the
problem is even greater in other, more-northern parts of the country.
Ninety-six patients between the ages of 19 and 55
with subacute (specifically, two to six months post injury) and chronic
(i.e., at least a year after injury) injuries were recruited. All
individuals had motor, complete injuries ranging from the cervical C3 to
thoracic T10 level; specifically, 43% and 57% had tetraplegia and
paraplegia respectively. Seventy percent were male, and 46% and 54% were
white and African-American, respectively.
Vitamin-D levels were assessed in both the summer
and winter, periods with greater and reduced vitamin-D production,
respectively. Even in the study’s southern location in summer, 65% and
81% of subjects with subacute and chronic injuries, respectively, had
subtherapeutic vitamin-D levels, predisposing them to a loss of bone
density and a multitude of other vitamin-D-aggravated problems. As
expected, the situation was even worse in winter with 84% and 96% of
subjects with subacute and chronic injury, respectively, having
subtherapeutic levels. Vitamin-D levels are believed to be higher in
those with acute injury due to the residual stores of the vitamin
accrued before injury. These stores are depleted over time because the
injured individual tends to get outside in the sun less and consume a
diet supplemented with less vitamin D (e.g., forgoing fortified milk)
due to concerns of developing kidney stones. Due to darker skin
pigmentation, vitamin-D deficiencies were greater in African Americans.
The investigators concluded “Vitamin D
insufficiency and deficiency are found in the majority of patients with
chronic SCI and in many with acute SCI. Initial screening for [vitamin
D] should be performed early in rehabilitation. Periodic monitoring in
the chronic setting is highly recommended.”
The problem of vitamin-D deficiency in individuals
with SCI was further documented by Dr. Gregory Nemunaitis and
colleagues (USA) in a 2011-published study. Specifically, vitamin-D
levels were assessed in 100 patients with SCI who were consecutively
admitted to acute inpatient rehabilitation. Of these patients, 93% had
inadequate levels of the vitamin, with 21% classified as severely
deficient.

GINKGO
BILOBA’S NEUROPROTECTIVE EFFECTS:
Obtained from the leaves of a large deciduous tree
originally from China, Ginkgo biloba is one of mankind’s most
ancient medicines. Fossil records indicate the species has been around
for over 200-million years, and some ginkgos at Chinese temples are more
than 1,500-years old. Given the trees are highly disease and insect
resistant and grow in urban environments where other trees can not, it
is not surprising that they possess substances with medicinal
properties.
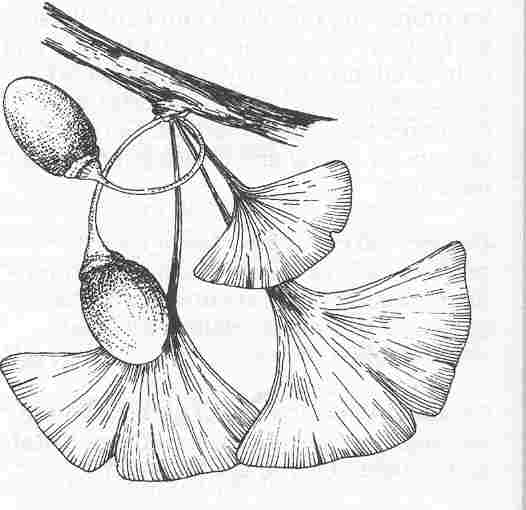
In Europe, ginkgo is the most widely sold and
prescribed plant-based medicine; in the U.S., it is one of the top ten
best-selling herbal remedies. Supported by varying degrees of animal
research and clinical studies, ginkgo may provide benefits for a variety
of disorders, including:
Ginkgo operates through several potential
physiological mechanisms especially relevant for neuronal health. For
example, it is an antioxidant, maintains cell-membrane integrity,
enhances oxygen use and metabolism, augments neurotransmission, and
inhibits a form of programmed cell death called apoptosis.
Using a rat model of acute injury, Turkish
investigators showed that ginkgo extract inhibits post-injury lipid
peroxidation, a biochemical process that mediates secondary damage to
the injured cord (Koc et al. Res Exp
Med 195, 1995). Ginkgo’s inhibition was even greater than
methylprednisolone (MP), a glucocorticoid-steroid drug which is now
routinely administered after injury to minimize neurological damage.
More recently, Chinese investigators demonstrated
that ginkgo extract is neuroprotective in rats with experimental SCI (Ao
et al. Spinal Cord, 44, 2006). Specifically, after cutting
the spinal cord in half at the thoracic T-9 level, rats were given
either ginkgo or saline. The ginkgo-treated rats had smaller
injury-related cavities, less conduction-inhibiting demyelination, and
less apoptotic neuronal cell death.

FASTING:
Recent paradigm-expanding research carried out by Drs. Ward
Plunet and Wolfram Tetzlaff and colleagues at the University of
British Columbia (Canada) suggests that fasting enhances nervous-system
regeneration after SCI. Specifically, rats with experimental cervical
injuries were randomized into two groups. The control animals had free
access to food and water, while the experimental animals received food
only every other day starting immediately after injury.
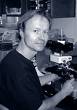
Compared to controls, fasted rats had improved gait
and forelimb function. Fasting also preserved neuronal integrity,
reduced the size of the injury-site lesion by more than 50%, and
increased sprouting of axons. Finally, the blood level of a
neuroprotective agent (called beta-hydroxybutyrate) increased 2-3 times
on the fasting days. A similar neuroprotective effect has also been
observed by other scientists for traumatic brain injury.
The investigators concluded that because
every-other-day-fasting “is a safe, non-invasive, and low-cost
treatment, it can readily be translated into the clinical setting of
spinal cord injury and possibly other insults.”

Buyang Huanwu
Decoction (BYHWD):
BYHWD is a Chinese herbal medicine that has been
used for centuries to treat a variety of disorders, including paralysis.
From a Traditional Chinese Medicine viewpoint, it’s used to “invigorate
the body, promote blood circulation, and activate meridians (energetic
channels).” The decoction is composed of extracts of a number of Chinese
herbs or remedies, including astragalus, dong quai, red peony root,
Rhizoma Chuanxiong (Lingusticum), earthworm, peach seed, and safflower.
Demonstrating that ancient wisdom often has much
contemporary validity, studies indicate that BYHWD, indeed, exerts some
neuroprotective and regenerative effects. For example, animal research
suggests that this herbal decoction can promote nerve regeneration after
stroke and both peripheral-nerve and spinal-cord injuries.
In the case of SCI, Dr. An Chen et al
(China) have evaluated BYHWD in a rat model of injury in which one side
of the cord was transected at the cervical level. After transection, the
rats were administered either the BYHWD or a distilled-water control for
eight weeks via gastrogavage (i.e., through a stomach tube). After this
time period, the number of surviving neurons on the cord’s injured side
for both BYHWD- and water-treated groups were compared to the neuron
level on the non-injured side (i.e., a baseline comparison). Compared to
the uninjured side, 78% of the neurons remained with the BYHWD-treated
rats compared to only 58% of the water-treated rats. In other words, the
BYHWD decoction reduced injury-related neuronal loss from 42 to 22%.
In addition, cell bodies of surviving neurons
atrophied by 64% in the water-treated controls compared with 35% in the
BYHWD-treated rats. In other words, BYHWD enhanced the apparent
robustness of the surviving neurons.
Especially significantly, only in the BYHWD-treated
rats did axons regenerate through the injury site. And, as would be
expected with such regeneration, these rats recovered more forelimb
function, the physical area affected by the experimental transection
injury.
In another study, Dr. Lihong Fan and
colleagues (China) evaluated the effects of BYHWD in a rabbit model of
SCI. In this model, injury was generated by temporarily shutting off
blood flow to the spinal cord’s lumbar region (i.e., ischemia),
affecting hind-limb function. The rabbits were treated with either BYHWD
or saline starting seven days before injury and continuing two days
after injury. Hind-limb function was then measured using a scale ranging
from 0 (complete paralysis) to 5 (normal function). Forty-eight hours
after injury, the BYHWD-treated rabbits averaged 3.4 on this scale
compared to 2.6 for the saline-treated controls.
With respect to peripheral nerve injuries, Dr.
Yueh-Sheng Chen et al (Taiwan) demonstrated that BYHWD stimulates
growth in regenerating nerves. In this study, a 10-millimeter gap was
created in the rat sciatic nerve (a nerve that runs down the leg from
the back) and then bridged by a silicon-rubber tube. Regeneration across
the gap was compared in BYHWD-treated rats and control animals who
received no BYHWD. Nerves regenerated across the gap in 89% percent of
the BYHWD-treated rats compared to only 70% of controls.
Although these BYHWD-related improvements may
appear modest, it is important to underscore that studies have shown
that substantial physical function can be retained even if only a
relatively small percentage of neurons survive the injury.
BYHWD may mediate its neuroprotective effects
through several physiological mechanisms. For example, scientists have
shown that BYHWD 1) stimulates the outgrowth and differentiation of
neurites on neuronal stem cells (neurites are processes budding out from
immature neurons, such as developing dendrites and
axons); 2) inhibits apoptosis – a post-injury, programmed cell death of
spinal-cord cells; and 3) decreases free radical generation and
associated lipid peroxidation, biochemical processes that
mediates secondary damage to the injured cord.

Quercetin
Quercetin is another commonly available nutritional
supplement that has been shown to reduce neurological damage in animals
after acute injury. Belonging to a family of molecules called
flavonoids, quercetin imbues coloring to many foods, including apples,
red onions, red grapes, tomatoes, raspberries and other berries, and
broccoli. Evidence suggests that it provides benefits in cancer,
prostatitis, heart disease, cataracts, allergies/inflammation, and
respiratory disorders.
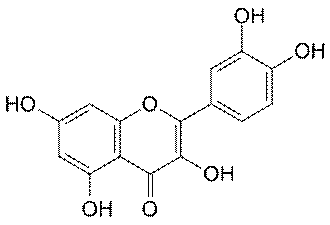
Quercetin
Like melatonin described previously, quercetin is
an antioxidant. By scavenging free radicals, it inhibits the
damage-perpetuating lipid peroxidation that occurs soon after injury. As
discussed above, this peroxidation impairs neuronal and
axonal membranes, resulting in further cell death.
Injury results in hemorrhaging.
This process causes the hemoglobin within red blood cells to
disintegrate, releasing oxidized iron. This reactive form of iron also
promotes nervous-system-compromising lipid peroxidation.
Quercetin has been shown to chelate or bind iron, preventing it from
reacting with the lipids on neighboring cells. In addition to its
antioxidant characteristics, quercetin has other properties that augment
its neuroprotective potential. For example, it is 1) lipophilic (i.e.,
affinity for fat or lipid), allowing it to diffuse through cell
membranes and scavenge free radicals within the cells, 2)
anti-inflammatory, and 3) anti-edematous, i.e., inhibits damage-causing
swelling.
Given such properties, Dr. E. Schultke and
colleagues (Canada) assessed the impact of treating injured rats with
quercetin. The rats were experimentally injured by exposing their cords
through a laminectomy and clipping them at the thoracic level for five
seconds. Different doses of quercetin or saline (i.e.,
control animals) were then injected into the body cavity (i.e.,
intraperitoneally) one hour after injury and every 12 hours thereafter
for either 4 or 10 days. Recovery of hind-limb function was evaluated by
a commonly used animal test called the BBB scale, which assesses
functional recovery on a scale from 0 (no hind-limb movement) to 21
(normal walking).
Although no saline-treated controls
walked, two-thirds of the quercetin-treated animals recovered some,
albeit compromised, walking ability. Supporting the hypothesis that iron
mediates damage, the tissue of the injured cords of
saline-treated-control animals tested positive for iron, but no iron was
detected in the cords of quercetin-treated animals.
The investigators reported the
results of a somewhat similar investigation in 2009. This study
evaluated the effects of different quercetin treatment regimens on
recovery in injured rats. Although no control animals regained
sufficient hind-limb function to walk, approximately 50% of the rats
treated with twice-daily doses of quercetin over three or 10 days were
able to walk. In general, the rats that were treated with quercetin for
a longer duration recovered more function. Compared to controls, more
spinal-cord tissue was preserved at the injury site in the
quercetin-treated rats.
The investigators have also
demonstrated quercetin’s potential neuroprotective effects in traumatic
brain injury, a disorder exhibiting injury mechanisms somewhat
comparable to SCI. In these experiments, rats with an experimentally
created brain injury were treated intraperitoneally (again, into the
body cavity) with either quercetin or saline one hour after injury and
thereafter at 12 hour intervals. The amount of neurological damage was
assessed by 1) electrophysiological measurements of nerve
activity/conduction and 2) various biochemical markers of oxidative
stress. These assessments indicated that quercetin-treated rats had less
neurological damage compared to the saline controls.

Vitamin E
Evidence indicates that the commonly consumed
nutritional supplement vitamin E may be neuroprotective after acute
injury. Vitamin E is a generic term for a class of molecules called
tocopherols, the most physiologically ubiquitous being alpha-tocopherol.
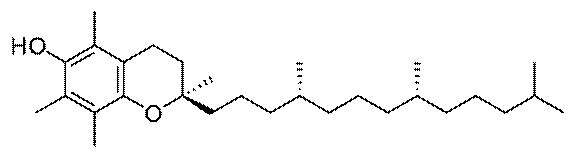
Alpha-tocopherol
form of vitamin E
Vitamin E is found in a variety of foods, including
vegetable oils, whole grains, dark green leafy vegetables, nuts and
seeds, and legumes. Vitamin-E supplementation may provide a number of
health benefits, such as promoting cardiovascular and eye health, and
preventing cancer and age-related cognitive decline.
Like melatonin and quercetin discussed above,
vitamin E is an antioxidant that protects cell membranes from the free
radicals generated through lipid-peroxidation. Research suggests that
vitamin E exerts its neuroprotective by inhibiting this damage-mediating
process after injury, and, by so doing, helps to preserve
neighboring neurons and axons.
Key studies include:
In 1987, Dr. Royal Saunders
and co-investigators (USA) reported the results of treating
experimentally injured cats with a combination of vitamin E (alpha-tocopherol)
and another antioxidant, selenium, on lipid peroxidation. Before injury,
cats were pretreated orally for five days with this combination.
Compared to untreated injured controls, the spinal-cord tissue at the
injury site of vitamin-E/selenium-treated cats had fewer molecules
associated with destructive, lipid-peroxidation. The investigators
concluded “the combination of alpha-tocopherol and selenium may protect
injured spinal cord tissue…by limiting these posttraumatic membrane
lipid changes.”
In 1988, Dr. Douglas Anderson
(photo) and colleagues (USA) evaluated the effect of vitamin E on
functional recovery in cats with experimental SCI. To produce the
injury, the lumbar area of the spinal cord was exposed by a laminectomy
and a compressing weight placed on it. The cats were treated orally with
vitamin E (i.e., alpha-tocopherol) for five days before and after
injury. Functional recovery was assessed by improvements in the cats’
ability to walk, run, and climb stairs.
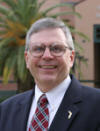
Four weeks post-injury, vitamin-E
treated cats recovered 72% of their pre-injury function compared to only
20% for similarly injured but untreated control cats. The investigators
concluded that “pretreatment with alpha tocopherol was extraordinarily
effective in promoting functional recovery in cats undergoing
spinal-cord compression.” However, they noted that because vitamin E
enters the central nervous system slowly, it must be administered before
injury, and, hence, is probably not a viable possibility for treating
SCI. (see Al Jadid study summarized below)
Reported in 1989, Dr. Kenichi
Iwasa and associates (Japan) compared the recovery of rats fed a
diet containing vitamin E at a level 25 times that fed to control
animals. The high vitamin-E diet was consumed for eight to ten weeks
prior to a compression injury produced by placing a weight on the
thoracic area of the exposed cord.
Hindlimb function was assessed
using a scale ranging from 0 (no voluntary movement) to 4 (complete
recovery). One day after injury, the vitamin-E-treated rats had a
hindlimb score of 3.5 compared to 2.4 for controls. In addition,
vitamin-E supplementation enhanced the recovery of nerve conductivity
and spinal-cord blood flow, and reduced the level of molecules
associated with lipid peroxidation. Finally, microscopic examination of
the injured cord tissue showed less damage, such as bleeding and edema,
in vitamin-E-treated animals. The researchers concluded that
“supplementation of the diets with vitamin E only had a dramatic effect
in preventing motor disturbance.”
In a somewhat similar study
reported in 1990, this investigative team compared recovery in rats fed
the aforementioned control diet with rats fed a vitamin-E deficient
diet (specifically, 20-times less). In other words, this study is
comparing controls to vitamin-E-deficient and not -supplemented animals.
The results indicated that vitamin-E-deficient rats 1) had less recovery
of hindlimb function, 2) less restoration of spinal-cord blood flow, 3)
more compromised nerve conduction, 4) more bleeding and edema, and 5) a
greater production of chemicals associated with lipid peroxidation.
Reported in a recent 2009 article, Dr. Al Jadid
and colleagues (Saudi Arabia) reconfirmed vitamin E’s neuroprotective
effects in rats with experimental SCI. Like previous studies, a
compression injury was produced by placing a weight on the cord after
exposing it with a laminectomy. Injured rats were divided into three
groups: a a saline-treated control group, and two groups that received
different levels of vitamin E.
Unlike earlier studies, apparently the rats were
not pretreated with vitamin E; supplementation was started at the time
of injury and continued for 14 days. This is a key difference in study
design because pretreatment is obviously not a real-world therapeutic
option. As mentioned above, because vitamin E was
thought to enter the central nervous system slowly, it was not
considered a viable candidate to administer after injury. This
assumption is apparently not correct as demonstrated by Dr. Al Jadid’s
research.
In this study, post-injury
functional recovery was measured using an activity cage, which uses
horizontal and vertical sensors to measure animal movements. In other
words, injured rats who have recovered more function would trigger the
sensors to a greater degree. Both vitamin-E supplemented groups rats had
statistically significant improvements in function by the end of the
study compared to controls. These results suggest that vitamin E,
indeed, may be a useful SCI treatment option.

Chinese Skullcap
One of the 50 foundation herbs of Chinese herbology,
Chinese Skullcap has been used to treat a multitude of ailments,
including epilepsy, hepatitis, infections, inflammatory diseases, and
cancer. Also known as Baikal Skullcap, Huang Qin, and by its scientific
name Scutellarai baicalensis, Chinese Skullcap should not be
confused with American Skullcap, which exerts different physiological
influences.
Chinese Skullcap
contains
a variety of biologically active molecules, including flavonoids.
Flavonoids, such as quercetin discussed before, provide the pigmentation
in many of the plant foods we eat. Because they are strong antioxidants,
scientists theorized that flavonoid-endowed Chinese Skullcap could
protect neurons from post-injury oxidative stress and damage-mediating
free radicals. Flavonoids contained within Chinese
Skullcap have been shown to cross the blood-brain barrier; hence, they
are able to get to the injured cord where they are needed.
Dr. Tae Yune
and colleagues (Korea) examined the effects of Chinese Skullcap in rats
with a contusion injury produced by dropping a weight on the exposed
cord (36). The injured rats were treated orally with varying levels of
Chinese Skullcap or water as a control beginning two hours after injury
and then once daily for 14 consecutive days.
Functional improvement was measured
by 1) the BBB locomotor score, which measures hind-limb functional
recovery on a scale from 0 (no hind-limb movement) to 21 (normal
walking); 2) the grid-walk test measuring foot-placement accuracy; and
3) footprint analyses after paws were dipped in dye. As measured by the
BBB evaluation, hind-limb functional recovery was significantly higher
in Skullcap-treated rats 14 to 35 days after injury. In addition, these
rats made fewer mistakes on the grid-walk test, and footprint analyses
demonstrated better forelimb-hindlimb coordination, including less toe
drag.
This improved function could be the
result of a number of physiological effects that Skullcap exerts on the
injured cord. For example, the investigators demonstrated that Skullcap
inhibits the production of inflammatory molecules involved in the injury
process, reduces oxidative stress, inhibits the programmed cell death of
neurons and their support cells (a process called apoptosis), decreases
the size of the injury-site lesion, and lessens the post-injury loss of
myelin, the insulating, conduction-promoting material surrounding
neurons.

Acetyl-L-Carnitine
The amino acid acetyl-L-carnitine is a frequently
used nutritional supplement.
It
is a chemically modified form of L-carnitine, a substance abundantly
found in red-meat and dairy products and also produced to some degree by
the brain, liver and kidneys. Unlike many substances, acetyl-L-carnitine
can pass through the blood-brain barrier and, by so doing, exert
physiological, neuroprotective effects on nervous-system cells. For
example, evidence suggests that acetyl-L-carnitine may be beneficial for
age-related neurodegenerative conditions, such as Alzheimer’s dementia,
memory difficulties, depression, etc.
Acetyl-L-carnitine also affects the functioning and
viability of mitochondria, all-important organelles within cells
responsible for generating the chemical fuel (i.e., ATP or adenosine
triphosphate) used to drive most biochemical processes. Mitochondrial
functioning can be severely compromised in injury-affected neuronal
tissue, which, in turn, contributes to the secondary injury processes
that magnify the damage after the initial traumautic insult.
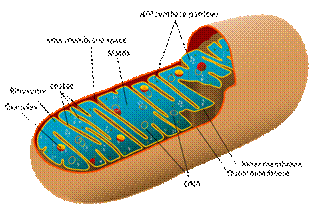
In a series of investigations in animal models,
Dr. Samir Patil and associates (USA) showed that acetyl-L-carnitine
helps to preserve mitochondrial function after SCI. As a consequence of
this preservation, there was less tissue damage at the injury site, and,
as expected with this observation, more function was retained.

Herbal Formulation JSK
Anecdotal reports
from China suggest that treatment with the JSK (Jiu-Sui-Kang) herbal
formulation after SCI may enhance functional recovery by improving
post-injury physiology and biochemistry. Although the specific
composition of the proprietary JSK herbal formulation has not been
disclosed, the formulation contains a number of Traditional Chinese
Medicine herbs, including Ginseng, Chuanxiong Rhizoma, Glycyrrhizae
Radix, Paeoniae Alba Radix, and Cinnamomi Cortex.
Dr. Caixin Su
and colleagues (Canada) investigated JSK’s therapeutic potential in a
commonly used rat model for acute SCI (ref). Rats injured by compression
were randomly divided into a treatment group administered a daily dose
of JSK solution or a control group receiving the same solution lacking
the JSK. The locomotor ability of all rats were periodically assessed
using the BBB locomotor rating scale which measures recovery of
hind-limb function on a scale from 0 (complete loss of function) to 21
(normal function).
Using this scale, it
was shown that JSK-treated rats had more preservation of function after
injury compared to control rats. Specifically, the JSK-treated rats
recovered to a 13.8 locomotor score compared to 11.2 for the controls, a
statistically significant difference. In addition, experiments
demonstrated that JSK-treated animals had 1) less tissue damage at the
injury site, 2) less injury-related loss of body weight, 3) less
injury-site deposition of fibrinogen, an inflammation molecule that
inhibits neurite outgrowth, 4) less injury-associated cell death by
apoptosis (defined in glossary), 5) a decreased production of Rho, a
molecule that inhibits axonal growth and regeneration (see Cethrin
discussion elsewhere), 6) more axonal sparing, including preservation of
the insulating myelin surrounding the axons, and 7) an increase in the
expression of blood-circulation-enhancing molecules.
The investigators
concluded that “JSK appears to target multiple biochemical and cellular
pathways to enhance functional recovery and improve outcomes of SCI.”
TOP