1) Melatonin
2) Estrogen
3)
Progesterone
4)
Testosterone
5)
Ghrelin

1)Melatonin: Readily available from vitamin stores and other
sources, melatonin is a key hormone produced by the pineal gland. Its
production is closely correlated to our sleep-wake cycle and is
specifically inhibited by light and stimulated by darkness. The pineal
gland converts the amino acid tryptophan into serotonin (a
neurotransmitter) and, in turn, melatonin. The melatonin then is
released into the bloodstream and cerebrospinal fluid, where it can
interact with cells throughout the body.
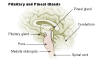
Through complicated neuroanatomical wiring,
photosensitive cells in the retina detect light and send signals to
structures that regulate our 24-hour circadian rhythms. These signals
then go out of the head to the cervical spinal cord, where are they are
routed back to the pineal gland. Hence, cervical, but not lower-level,
injuries will compromise the pineal gland and its melatonin production.
Called the sleep hormone, melatonin is used as a
sleep-aid for insomniacs, shift workers, and jet-lagged travelers.
Because it has been extensively consumed, it is presumably reasonably
safe. This is important because animal studies suggest that melatonin is
neuroprotective after acute injury. Although we need to be cautious in
extrapolating the results of animal studies to humans, its extensive use
makes it a better therapeutic candidate for acute injury in humans.
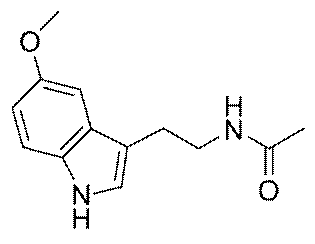
In addition to its hormonal action, melatonin is a
powerful antioxidant that protects cells from damaging oxidation.
Specifically, it is a highly efficient scavenger of free radicals,
which, because they possess an unpaired electron, seek out another
electron to achieve a more stable energetic state. Melatonin’s
lipophilic structure (i.e., affinity for fat or lipid) allows it to
diffuse through the membranes surrounding cells and scavenge free
radicals inside the cell.
After the initial mechanical injury
in SCI, a complicated physiological chain reaction generates
free-radicals, which steal electrons from the lipids in cell membranes.
Called lipid peroxidation, this process impairs neuronal and
axonal membranes, resulting in further cell death.
Like the frequently administered
methylprednisolone, animal studies indicate that melatonin inhibits
lipid peroxidation and various injury-aggravating inflammatory
processes. Sample studies include:
Dr. Toru Fujimoto
and colleagues (Japan) examined melatonin’s neuroprotective effects in
rats with experimental SCI. The spinal cords were injured at the T12
level by the pressure of a weight placed on the exposed cord. Melatonin
was injected into the body cavity (i.e., intraperitoneally) at 5
minutes, and 1, 2, 3, and 4 hours after injury. Saline was injected into
control rats. Because the amount of injected melatonin was much higher
than endogenously (i.e., produced from within) generated levels,
background levels were not considered experimentally relevant (albeit,
see Ates, et al below). Compared to controls, the melatonin-treated rats
had less lipid peroxidation, smaller injury-site cavities, and retained
more hind-limb function.
Dr. S. F. Erten
et al (Turkey) assessed melatonin’s effects in rabbits
with spinal-cord ischemia produced by clamping down on blood vessels
leading to the cord. Melatonin was intraperitoneally introduced either
10 minutes before or after clamping. The melatonin-treated rabbits had
less lipid peroxidation.
Dr. Jin-bo Liu
and associates (China) examined melatonin’s neuroprotective effects in
rats with injuries produced by dropping a weight on the exposed spinal
cord and dosing them intraperitoneally with melatonin. The investigators
concluded that “melatonin can prevent oxidative damage, reduce
neurological deficit, and facilitate the recovery from spinal cord
injury.”
Drs. Tiziana Genovese
and colleagues (Italy) provided further evidence of melatonin’s
neuroprotective effects. In their experiments, injury was produced in
rats by clipping the exposed spinal cord. Melatonin was administered
once before clipping and several times afterwards. The investigators
concluded “that melatonin can exert potent anti-inflammatory effects”
and enhanced hind-limb functional recovery.
Dr. Suleyman Cayli et al
(Turkey) compared the effectiveness of 1) melatonin, 2) the commonly
used methylprednisolone, and 3) a combination of the two drugs. After
injury was produced in rats by dropping a weight on the exposed cord,
the drugs were injected intraperitoneally, and various assessments
carried out over time. Compared to controls, improvements were noted in
all three treatment groups, including enhanced neuronal conduction,
recovery of motor function, decreased injury-promoting lipid
peroxidation, and improved injury-site structural integrity. The
combination treatment of melatonin and methylprednisolone was best at
inhibiting lipid peroxidation.
Earlier, it was implied that the
endogenous levels of melatonin produced by the body did not play a
significant neuroprotective role after SCI. However, research by Dr.
O. Ates and colleagues (Turkey) suggest that physiological
background levels may, indeed, be quite important. In addition to
looking at the neuroprotective properties of externally administered
melatonin, the investigators assessed the effect of removing the rat’s
pineal gland and, hence, the body’s melatonin source before injury. Such
pinealectomy increased the amount of lipid peroxidation after injury.
The investigators concluded: “These findings suggest that reduction in
endogenous melatonin after [pinealectomy] makes the rats more vulnerable
to trauma…”
These findings actually have
considerable relevance to humans. Specifically, for a variety of
reasons, including environmental, pineal functioning tends to
diminish over time. In adults, melatonin-compromising calcification of
the pineal gland is not uncommon, a process in which gritty deposits
called brain sand accumulate in the gland. It suggests that individuals
with such calcification will have more neurological damage after injury.
In 2012, Dr. M. Ersahin’s investigative team
(Turkey) evaluated melatonin’s potential to preserve bladder function in
rats experimentally injured at the thoracic T10 level. Results indicated
that “melatonin reduces SCI-induced tissue injury and improves bladder
functions through its effects on oxidative stress” and nerve growth
factor.
In 2012, Dr. S. Park and colleagues (Korea)
reported that in rats with SCI produced by contusion “both endogenous
and exogenous melatonin contributes to neural recovery and to the
prevention of skeletal muscle atrophy, promoting functional recovery
after SCI.” The investigators concluded that the study “supports the
benefit of endogenous (i.e., produced by the body) and use of exogenous
melatonin as a therapeutic intervention for SCI.” Earlier, these
investigators investigated the impact of treating injured rats with a
combination of melatonin and exercise13). The results indicated that the
combined therapy reduces the amount of secondary damage after injury.

2) Estrogen: Although
estrogen exerts many physiological effects in both women and men, it is
most well known as the female sex hormone. In women, estrogen is
primarily produced by the ovaries. It regulates the female estrous or
reproductive cycle, and promotes the development of secondary sexual
characteristics. Men also produce the hormone but at a much lower level.
In men, the hormone is synthesized by the testis and plays a key role in
testicular function.
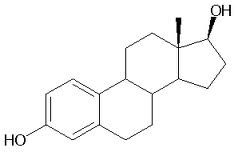
Estrogen derivatives are a key component of many
oral contraceptives and also have been used for postmenopausal
hormone-replacement therapy. In men, estrogen has been employed to treat
prostate cancer. Although estrogen’s reproductive roles receive the most
attention, this potent multiactive hormone can influence diverse
physiological processes. As such, it theoretically has broad therapeutic
potential much beyond its more obvious roles, including as a possible
protective agent after neurotrauma.
The SCI neuroprotective possibilities have been
extensively studied by Dr. Naren Banik and colleagues at the University
of South Carolina using animal models of SCI, as well as cultures of
neuronal cells.
Animal Studies: SCI was produced by
accessing the thoracic spinal cord of rats through laminectomy and
dropping a weight on the exposed cord. Essentially, this is an
experimental version of the sort of contusion injury experienced by many
individuals with SCI. The rats were then treated intravenously with
estrogen 15 minutes and 24 hours after injury, and, for the next five
days, with a single daily dose injected into the body cavity. Recovery
of locomotor function was followed for six weeks, and the amount of
improvement observed compared to similarly injured control rats which
received no estrogen.
Locomotion was assessed using the BBB scale, a
commonly used animal test which measures recovery of
hind-limb function on a scale from 0 (no hind-limb movement) to 21
(normal walking). At the end of the observation period, the average BBB
score for the estrogen-treated rats was 13 compared to nine for the
controls. Functionally, these statistically significant differences mean
that when compared to controls, the estrogen-treated rats were better
able to support their body weight, make weight-supported steps, and
coordinate hindlimb/forelimb stepping. The investigators concluded that
“estrogen treatment significantly increased the locomotor function in
the injured animals over the 42-day postinjury period…”
Possible Mechanisms: These investigators and
others have devoted much effort trying to understand the specific
biological mechanisms by which estrogen mediates neuroprotection. The
damage-spreading, pathophysiological cascade after the initial physical
insult is extraordinarily complex and is the reason why SCI has been
difficult to understand at a molecular level. Given this complexity, as
well as estrogen’s increasingly documented, powerful multifaceted role
in the body, there are many possible biological systems in which it
could target. Some of the possibilities are briefly highlighted below.
It is emphasized, however, that these are often complex interlinked and
interdependent processes.
1) Calcium Influx: Neuronal conduction
depends upon the right balance of calcium ions between the cell inside
and outside. Normally, there is a lot of calcium outside of the neuron
and relatively little inside. Injury disrupts the equilibrium, allowing
excessive calcium to flow into the cell. This influx initiates a
neural-destructive cascade that damages other neurons. By inhibiting
the calcium influx into the cells, estrogen lessens this
damaging-perpetuating cascade.
2) Apoptosis: Cells at the injury site die
of necrosis, while cells surrounding the site often die from apoptosis,
a form of secondary cell death in which a programmed sequence of events
leads to cell elimination. As a crude analogy, necrotic cell death is
like a quick death from being shot and apoptotic cell death is more like
a lingering death from cancer. Because apoptosis is potentially
reversible, treatments that turn this process around should help
minimize postinjury cell degeneration. By modulating the activity of
certain enzymes that promote postinjury apoptosis, estrogen slows down
degeneration.
3) Excitotoxicity: Routinely, certain amino
acids, like glutamate, are released from a pre-synaptic neuron and flow
to a nearby post-synaptic neuron, promulgating the nerve impulse.
However, after injury, cells burst, releasing too much glutamate.
Through interactions with receptors on neighboring cells, this excessive
glutamate will initiate a neurotoxic biochemical cascade. Estrogen
protects against this excitotoxicity-caused cell death.
4) Edema: Fluid accumulation at the injury
site creates damaging edema swelling. Estrogen-treated rats exhibit less
edema.
5) Inflammation: Inflammatory cells
infiltrate into the lesion area, which promotes secondary cell death.
Estrogen treatment lessens this infiltration.
6) Myelin: The fatty insulation surrounding
axons, myelin enables neurons to propagate a signal. SCI often results
in axonal demyelination, another process which is attenuated estrogen.
7) Blood Flow: Injury compromises
regeneration-promoting blood flow, contributing to secondary cell death.
Estrogen promotes the growth of new blood vessels (called angiogenesis),
enhancing postinjury blood flow.
8) Antioxidant: After the
initial mechanical injury in SCI, free-radicals are generated. Called
lipid peroxidation, these free radicals can steal electrons from
neighboring cell membranes, resulting in further cell death. A potent
antioxidant, estrogen may reduce free-radical-induced oxidative stress.
Given these findings and the fact that women have
much higher levels of estrogen than men, it is interesting note that
studies suggest that women actually recover more function after
neurotrauma.

3) Progesterone: Like estrogen, progesterone also appears
to provide neuroprotection. Although called the “pregnancy hormone,” it
is also synthesized to a lesser degree by men.
Progesterone is synthesized from cholesterol by the
ovaries, adrenal glands, and placenta. In the menstrual cycle,
progesterone levels are relatively low before ovulation (i.e., release
of a ripe egg from the ovary) and elevated afterwards. Progesterone
levels are much higher throughout pregnancy, drop to low levels after
birth and during lactation, and recede after menopause. In men, this
female-associated hormone is produced by the testes and, paradoxically,
is the biochemical precursor to the defining male hormone testosterone.
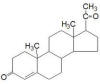
Progesterone exerts many biological influences
throughout the body above and beyond its more well-known effects in
reproduction, in part by affecting the expression of other
body-regulating hormones. Overall, our optimal functioning is dependent
upon a complex, interacting hormonal milieu, whose composition is
dependent on many factors, including gender, age, diet, life style, and
overall health.
Neurosteroid: With paradigm-expanding
implications, progesterone is also produced by and influences the
nervous system and, as such, has been termed a “neurosteroid.” Due to
this localized synthesis, nervous-tissue progesterone levels are not
necessarily a function of plasma levels of the hormone produced by more
traditional sources. Neurons and neuronal support cells (called glia)
actually have unique progesterone receptors on their outer membrane
surface. Like a key fitting in a lock, progesterone’s interactions with
these receptors can initiate complex, nervous-system-unique biological
responses. Although these responses are only beginning to be understood,
they seem to enhance neuronal health and viability.
Many studies suggest that progesterone treatment is
neuroprotective after trauma by limiting the loss of neuronal tissue
and, as a consequence, preserving function. Because membrane-soluble
progesterone can readily diffuse cross the blood-brain-barrier, unlike
many drugs, externally administered progesterone has the ability to
reach the nervous system. As a result, it has the opportunity to
interact with the various progesterone receptors on neuronal cells,
shifting the nervous-system environment to potentially a more
neuroprotective mode.
Although we must be careful in extrapolating
results to humans, progesterone neuroprotection has been documented in
numerous animal models of various neurological disorders, including
traumatic brain injury (TBI) and spinal cord dysfunction, such as SCI,
multiple sclerosis (MS) and amyotrophic lateral sclerosis (ALS). For
example, in animal models of MS, progesterone treatment lessens disease
severity, reduces inflammation, and restores the conduction-promoting
insulating myelin sheath surrounding neurons (see below). In ALS animal
models, the hormone inhibits the degeneration of motor neurons
TBI: Probably the most research has been
directed to TBI, partly because early studies suggested gender
differences in recovery after injury. For example, reproductive-cycling
female rats with high progesterone levels have less post-injury cerebral
edema (swelling) than male rats with inherently low progesterone.
Pseudopregnant rats (a pregnancy-like condition), whose progesterone
levels are especially high, had little post-injury edema.
Several clinical trials have examined
progesterone’s neuroprotective potential in humans. In the first,
Emory University investigators (USA) evaluated outcomes in a 100
subjects injured within the previous 11 hours treated with either
intravenous progesterone or placebo for three days. Thirty-days post
injury, progesterone treatment when compared to controls 1) reduced
mortality in the severely injured, and 2) and improved functional
outcomes in the moderately injured.
In a second trial, Chinese researchers randomized
159 patients with severe TBI sustained within the previous eight hours
to receive five days of either progesterone or placebo injections
intramuscularly. Six months later, progesterone-treated patients showed
greater functional improvement and lower mortality.
SCI; Research findings in TBI often, but not
always, have relevance to SCI. Although human studies are lacking,
extensive SCI-focused animal research builds a strong case in support of
progesterone’s neuroprotective potential for SCI. Given the complex
physiological cascade that occurs after injury, there are many
interacting, biological processes that progesterone could target. For
example, studies suggest that post-injury progesterone treatment:
·
Increases levels of growth factors that enhance neuronal
survival and axonal regeneration.
·
Restores the expression of enzymes involved in the
transport of sodium and potassium ions across the membranes of neurons -
a process needed for neural transmission.
·
Protects damaged neurons from cell death and the
disintegration of neuron ultrastructure.
·
Protects neurons from toxic levels of amino-acid
neurotransmitters that have been released from nearby damaged cells.
·
Reduces inflammation by decreasing various cells and
molecules involved in the inflammatory response.
·
Lessens damage-spreading fluid accumulation or edema.
·
Inhibits oxidation from injury-created
free-radicals, which steal electrons from and, as a result, damage
neighboring cell membranes.
Remyelination: In
addition to the aforementioned, evidence indicates that progesterone
promotes the post-injury remyelination of neuronal axons. Myelin is the
fatty insulating material enveloping axons, i.e., the fibers that
conduct electrical impulses away from the neuron’s cell body to other
nerves or muscles. When axons are demyelinated, channels between the
inside and outside of the axon are exposed, in turn, causing disruption
in the ionic equilibrium needed for neural transmission. Although most
associated with MS, demyelination frequent occurs after SCI. Intact
neurons may still traverse the injury site, but because they have lost
their insulation, they no longer conduct. In theory, therapies that help
restore the myelin sheath should re-establish some function-restoring
conduction.
In the spinal cord, myelin is produced by
oligodendrocytes, a neuronal support cell which is formed through
the differentiation of oligodendrocyte precursor cells. Because
oligodendrocytes are extremely sensitive to injury, much-needed
remyelination capability is lost. Evidence indicates that progesterone
treatment enhances the proliferation of the normally quiescent precursor
cells into mature, myelin-producing oligodendrocytes, enhancing the
conduction of injury-waylaid neurons.
It is important to note when discussing potential
restorative treatments, only a relatively small percentage of intact,
functioning neurons are needed to regain significant function. In other
words, if progesterone-triggered remyelination can jump-start a few
neurons, significant function may accrue.
Hindlimb Functional Recovery: In spite of
this promising research, animal studies directed toward recovery of
function after SCI are limited and ambiguous in results. In one study,
Dr. Ajith Thomas and colleagues (USA) evaluated the effect of
progesterone treatment in rats with SCI produced by contusion, the sort
of injury common in humans. After injury, rats received progesterone
injected into the body cavity periodically for five days.
Compared to controls, six weeks after injury,
progesterone-treated animals recovered more function and
had more tissue preservation at the injury site.
However, research by Dr, Dominic
Fee et al (USA) could not replicate these benefits. Specifically, after
an experimental contusion injury, rats were treated with progesterone
with several dosing regimens for up to 14 days. Three weeks after
injury, no significant improvement in hindlimb function was observed
between the progesterone-treated and control animals.

4)
Testosterone: Testosterone is primarily produced by the
testes in men and, to a lesser degree, the ovaries and placenta in
women. Small amounts are also produced by the adrenal glands. In men,
testosterone promotes 1) the development of reproductive tissue, sex
organs, and secondary sexual characteristics such as body hair and voice
deepening (i.e., androgenic role); and 2) sexual function, growth of
muscle mass and strength, and bone density (i.e., anabolic influence).
The second benefit also makes testosterone important in women.
Testos
terone
is synthesized from cholesterol, which is an essential biochemical
building block for many hormones and nervous-system molecules. Its
production is regulated by the hypothalamic-pituitary-testicular axis, a
tongue-tying description for a regulatory, feedback loop used by our
bodies to attain hormonal balance.
Briefly, the hypothalamus, a region of
the brain located above the brain stem, regulates the release of key
hormones by the nearby pituitary gland, which then stimulates testicular
cells to produce testosterone. However, as testicular production
increases, the elevated testosterone levels start shutting off the
brain’s release of testosterone-stimulating molecules. As a result,
testosterone output decreases (figure). Because testosterone synthesis
is central-nervous-system-driven process, a major CNS disruption like
SCI can affect testosterone levels.
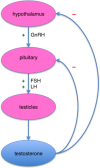
Carried via the bloodstream, the testicular-synthesized testosterone (or
its derivatives) reaches the target tissue, such as muscle, bone, sex
organs, kidney, liver, and brain. It is then transported into the cells
and interacts with the DNA of specific genes. This interaction cranks-up
gene expression and, in turn, the tissue products resulting from that
expression - e.g., more muscle, etc. As a simple analogy, it’s like
speeding up a manufacturing assembly line.
Normal testosterone blood levels range from about
300-1,000 and 25-90 nanograms per deciliter in men and women,
respectively (nanogram is one-billionth of gram; deciliter is one-tenth
of liter).
Only about two percent of the body’s testosterone
is biologically active free testosterone. The remaining
testosterone is either 1) bound to albumin, a carrier protein in
the blood plasma (yet still bioavailable), or 2) complexed with sex
hormone binding globulin (SHBG) (no longer bioavailable). To give a
better idea of one’s true testosterone status, laboratory assessments
should measure both total and free testosterone.
Low testosterone levels are referred to as
hypogonadism, a condition associated with osteoporosis (loss of bone
density), decreased lean body mass (i.e., more fat), less strength,
reduced mental acuity and focus, mood changes, fatigue, less sexual
desire, and erectile dysfunction. As men age, testosterone levels
decline, a process called andropause after middle age.
In addition to age, various factors contribute to
low testosterone levels. For example, 1) excessive amounts of the
hormone can be converted into estrogen, 2) as men age or become sick,
more testosterone is taken out of commission by binding proteins, 3) the
pituitary and hypothalamus may not release sufficient hormones to
adequately stimulate testicular testosterone production, 4) the
testicles may have lost their ability to generate testosterone, and 5)
medications may suppress production.
Testosterone Replacement Therapy (TRT):
Although once the realm of body-building athletes, many have adopted TRT
to mitigate the consequences of testosterone diminution from aging or
other causes, such as SCI (87). TRT-related benefits potentially include
less osteoporosis, type-2 diabetes, cardiovascular disease, erectile
dysfunction, depression and anxiety, and Alzheimer’s disease.
TRT requires ongoing monitoring to manage potential
side effects. Because testosterone influences many bodily functions, it
should be prudently used. TRT should be viewed as a long-term commitment
to not only the therapy but various medical assessments that should be
carried out on an ongoing basis. TRT will shut down testicular
testosterone production. By taking testosterone, you will disrupt the
aforementioned hypothalamic-pituitary-testicular feedback loop and turn
off whatever limited synthesis you had before treatment. As a result,
if you have to discontinue TRT for any reason, your body will be
generating little testosterone, and your physical and mental state will
reflect this paucity. The body probably will eventually recover to
baseline levels, but it may take a while.
SCI & Testosterone (88-100) SCI is
correlated with many of the problems associated with low testosterone.
For example, depending upon the injury, 1) skeletal muscle mass
atrophies by 30-60%, and 2) bone loss continues at an enhanced rate for
decades (88). If injury, indeed, compromises testosterone production and
that disruption hastens post-injury bone and muscle loss, it becomes an
extraordinarily important issue to study, as well as approaches, such as
TRT, that may promote function-enhancing hormone levels.
The results of earlier studies were ambiguous due
to potential confounding factors, such as participant age, time since
injury, and injury level or completeness. For example, if one study
focused on the acutely injured and another on the chronically injured,
results could be different; or if a study didn’t consider such a factor,
individual results could offset each other. As investigations better
controlled these factors, it has become evident that SCI compromises
testosterone levels for many individuals after injury. Several sample
studies are summarized below:
1) University of Missouri scientists
(USA) have carried out a series of studies evaluating testosterone
levels after SCI. In 2006, they examined testosterone levels in 92 men
with SCI admitted to inpatient rehabilitation (94). Averaging 39 (range
19-92) years old, the injuries were roughly evenly divided between
paraplegia and quadriplegia injuries, and complete and incomplete
injuries. All had sustained their injuries within the past 15 years.
Although most guidelines define low testosterone to be below 300 ng/dl [nanogram
is one-billionth of gram; deciliter is one-tenth of liter], the
investigators used 240 ng/dl as a cutoff point, a level they called
abnormally low.
Overall, 83% had levels below this threshold. Men
with more acute injuries (< 4 months) averaged only 160 ng/dl. Given
testosterone’s important body-maintenance role, these are shockingly low
levels that inevitably compromise recovery efforts. Statistically, the
odds for having low testosterone for men with acute versus chronic
injuries were 6.7-times greater. Although testosterone differences were
noted between paraplegia and quadriplegic injuries and complete and
incomplete injuries, the study was not large enough to demonstrate
statistical significance.
2) Reported in 2008, the same group
evaluated testosterone levels in 102 men recruited from rehabilitation
facilities (92). Average age was 46 (range 18-82). Testosterone levels
averaged 220 ng/dl, with 60% of the subjects having abnormally low
hormone levels (i.e., < 240 ng/dl). As before, men with more acute
injuries were more likely to have low testosterone. Specifically, 69% of
individuals in the acute-injury phase (<4 months) had low testosterone
compared to only 40% of those in the chronic phase (12+ months).
3) Also in 2008, the investigators reported
the results of treating 50 men with TRT recruited within several weeks
of injury at an inpatient rehabilitation facility (93). All had low
testosterone levels (averaging 136 ng/dl) and were given monthly,
intramuscular injections of the hormone. Because there was no control
group, the investigators compared motor recovery of their subjects with
the outcomes of 480 non-testosterone-treated men who were included in a
national SCI database. This comparison suggested that TRT promoted
strength gains in those men with incomplete injuries already having
residual muscle preservation.
4) Because only a small percentage of total
testosterone is biologically active, Dr. Berna Celik and
colleagues (Turkey) assessed both total and free testosterone levels in
44 men with SCI recruited from an inpatient rehabilitation unit (91).
The men averaged 35 (range 16-71) years old, and possessed a spectrum of
complete and incomplete injuries at various neurological levels.
Twenty-seven and 17 subjects had been injured less and more than one
year, respectively. The results indicated that both total and free
testosterone was lower in the group who had been injured less than a
year. In this study, no correlation was found between testosterone
levels and function as assessed by the Functional Independence
Measure (FIM - a predictor of one’s overall ability to perform
activities of daily living).
5) Dr. William Bauman and colleagues (USA)
have initiated a clinical trial to examine TRT’s potential benefits in
11 men with chronic SCI with low circulating levels of
testosterone compared with 11 men with normal levels of the hormone. The
subjects who had low levels of testosterone were administered a
testosterone patch daily to return testosterone level to the normal
range and, in turn were sequentially evaluated for possible changes in
body composition, energy expenditure, and other factors.
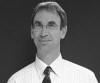
Preliminary results were reported in 2011.The
subjects averaged 43 years old in the treatment group and 35 years old
in the control group with average durations of injury for these groups
of 12 and 13 years, respectively. In the treatment group, eight subjects
had complete injuries and three incomplete injuries. In the control
group, nine subjects had complete injuries and two incomplete injuries.
After a six-month baseline period, TRT was provided for 12 months, after
which there was a six-month washout period in which no hormone was
administered.
The findings were that 12 months of TRT
significantly improved lean tissue mass (i.e., more muscle) and
increased resting energy expenditure (the amount of calories the body
burns during rest is also an indicator of an increased total muscle
mass). These favorable changes have the potential to improve physical
function and general health in men with SCI and low circulating
testosterone levels.Neuroprotection: As discussed previously,
much evidence suggests that estrogen and progesterone can be
neuroprotective after SCI. Both inhibit a variety of neuron-damaging
processes that occur after SCI and, by so doing, may limit neuronal
tissue loss and preserve function. More limited evidence suggests that
testosterone may also exert some neuroprotective role for a variety of
nervous-system disorders, including Alzheimer’s disease, ALS
(Amyotrophic lateral sclerosis), and perhaps SCI (95).
Testosterone can cross the blood-brain barrier,
meaning it can actually get to the target neurons. Furthermore, like a
sort of testosterone-specific Velcro, these neurons have receptors that
selectively bind the hormone. This binding can potentially trigger a
shift towards regenerative physiology. For example, studies have shown
that testosterone can increase neuronal differentiation, the outgrowth
of neurites (projections like axons and dendrites), cell-body size,
formation of synapses (connections) between neurons, and plasticity
(processes by which the nervous system returns to normal function).
In the case of SCI, studies indicate that
testosterone inhibits a damage-perpetuating excitotoxicity that occurs
soon after injury. Basically, after injury, damaged neurons release an
excitatory amino acid called glutamate, which can reach toxic
concentrations. Through interactions with receptors on neighboring
cells, excessive glutamate will initiate a neurotoxic biochemical
cascade. Apparently, testosterone can protect the spinal cord against
such damage.

5)
Ghrelin: Ghreline
is a 28-amino-acid peptide produced by a various body tissues,
especially the cells in the upper portion of the stomach. Increasing
before meals and decreasing afterwards, ghrelin is an
appetite-enhancing, hunger-stimulating hormone. Although its biology is
not well understood, ghrelin interacts with numerous tissues throughout
the body, including the central nervous system, suggesting the hormone
plays important physiological roles. In the case of the spinal cord,
ghrelin complexes with receptors found on the surfaces of neurons and
oligodendrocytes, neuronal support cells which produce the insulating,
conduction-promoting myelin surrounding axons.
Recent studies indicate that ghrelin may be
neuroprotective after injury by preserving tissue integrity and, as a
result, some function (58-60). For example, Dr. Jee Lee and
colleagues (South Korea) reported that ghrelin administration improved
hind-limb locomotor function in rats experimentally injured by
contusion. Their results indicated that this preservation of function
was probably due to ghrelin’s ability to inhibit the post-injury death
of neurons and oligodendrocytes (a process called apoptosis). This
inhibition reduces the size of the injury-induced spinal-cord lesion,
preserving axons, as well as the insulating myelin that surrounds them.
The investigators concluded that “ghrelin may represent a potential
therapeutic agent after acute SCI in humans.”
TOP