1) Introduction
2)Therapeutic
Exercise
3)
Standing/Ambulation
4) Manual Grasping
Control
5)
Bladder/Bowel Management
6) Respiratory Support
7) Epidural
Electrical Stimulation
1)
Introduction: Functional Electrical Stimulation (FES) uses low
levels of electrical current to stimulate physical or bodily functions
lost through nervous system impairment (1-7). FES is applied to
peripheral nerves that control specific muscles or muscle groups.
Because FES is an involved area with extensive
history, the summaries below provide only a superficial overview of the
technology. Various FES applications have moved to the forefront as they
evolved and then receded in priority. This ebb and flow will undoubtedly
continue in the future. As such, readers interested in learning more
about the subject are encouraged to consult the referenced resources.
FES is not a cure but a tool to regain specific
functions. Although in some cases FES can promote limited functional
recovery, it does not repair or regenerate the damaged spinal cord. FES
is ineffective if target muscles become denervated, which can be slight
or extensive, depending on the nature of the injury.
FES applications include standing, ambulation,
cycling, grasping, bowel-and-bladder control, male sexual assistance,
and respiratory control. Potential benefits include improved venous
return from lower limbs, osteoporosis prevention, fewer urinary
infections, muscle mass retention, and cardiovascular health.
Psychological benefits can result from improved functionality and
greater independence.
FES components include an electronic stimulator, a
feedback or control unit, leads, and electrodes. Electrical stimulators
can have one or multiple channels (outputs), which are activated in
unison or in sequence to produce desired movements.
Therapist-operated FES systems use switches or
dials to control activation. Control mechanisms for subject-controlled
FES include joysticks, buttons, switches, joint positions sensors, heel
switches, sip-and-puff devices, EMG electrodes, and voice activation.
Subject-controlled FES can be open- or closed loop.
In open-loop FES, the electrical stimulator controls the output.
Closed-loop FES employs joint or muscle position sensors to facilitate
greater responsiveness to muscle fatigue, or to irregularities in the
environment.
Electrodes act as interfaces between the electrical
stimulator and the nervous system and can be external (surface) or
surgically implanted depending on the application, device, and the
patient's needs.

2)
Therapeutic Exercise: Individuals with SCI can suffer
further health impairment through the chronic lack of physically
balanced exercise. FES-assisted therapeutic exercise (TE) can help in
this regard.
FES TE routinely uses ergometers of some sort
(e.g., stationary cycles, hand cranks, rowing devices) to exercise upper
or lower extremities. Physical benefits include improved cardiac output,
peripheral venous (blood) return, and muscle oxidative capacity. FES TE
can decrease spasticity, while increasing plasma endorphins, muscle
bulk, range of motion, and bone mineral density. Furthermore, it
can improve glucose tolerance and insulin sensitivity, cortisol levels,
wound healing, and self image.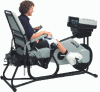
FES TE devices
include 1) the ERGYS 2 system, 2) the RT300
motorized FES ergometer, 3) Concept 2 indoor rowing machine, and
4) the BerkelBike, a hybrid
between a recumbent bike and a hand-cycle.

3)
Standing/Ambulation:
In addition to the physical effects of exercise, FES for standing,
transfer, and ambulation provides functional and psychological benefits.
Potential benefits include improved digestion, bowel-and-bladder
function, retardation of bone-density loss, decreased spasticity,
reduced pressure-sore risk, improved cardiovascular health, and improved
skin and muscle tone. FES-assisted ambulation allows greater access to
inaccessible locations and facilitates face-to-face interactions.
Systems
for standing and ambulation can be strictly FES, or combine FES with
various braces, including foot-and-ankle, knee, and long-leg braces. FES
standing or ambulation systems use walkers, parallel bars, or elbow
canes for balance and support. Depending on the system being used and
its application, physical requirements and contraindications can vary:
 | Upper
extremities needed for balance and support. |
 |
Intact lumbar and sacral spinal cord so stimulation can reach target
muscles. |
 | Trunk
stability for support and control. |
 | Arm
strength to use walker. |
 |
Commitment to intensive training and consistent use. |
 |
Sufficient finger or voice control to select menus. |
 | No
cardiac or respiratory problems. |
 | No
history of long-bone stress fractures, osteoporosis, or severe hip
or joint disease. |
 | Due
to effort involved in FES, not pregnant. |
 | No
severe scoliosis. |
 | No
morbid obesity. |
 | No
irreversible contractures. |
 | No
stimulation-preventing skin problems at stimulated sites. |
The physical
effort of FES-assisted ambulation is six to eight times that of
able-bodied walking. For this reason, less than five percent of FES
users walk more than 1,500 meters without rest, and, therefore, FES
ambulation is generally not a practical replacement for wheelchairs.
A well -known
FES-standing/ambulation system the Parastep® stimulates the
quadriceps muscles for leg extension, the peroneal nerve for hip
flexion, and the paraspinal muscles (or the gluteus maximus) for trunk
stability. Its belt-attached stimulator connects via wire leads to
self-adhesive electrodes. Fairly effort- and time-consuming training
programs are recommended for new users.
Over the
years, the Parastep system has been the focus of numerous studies,
including the following: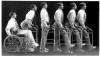
1) Dr. P.
Gallien and colleagues
(France) evaluated Parastep’s ambulation-fostering potential in 13
individuals (11 men, 2 women) with clinically complete injuries ranging
from the T4-T10 thoracic level. Age ranged from 17 to 42 years (average
27), and the time since injury varied from 5 to 240 months (average 5
years). Motor vehicle accidents caused all but one injury. After 30 or
less two-hour training sessions three to five times a week, 12 of the 13
subjects were able to ambulate. Walking distance averaged 76 meters.
Although one individual obtained 350 meters, only three exceed 100
meters. Walking speed averaged 0.2 meters/second (normal walking speed ~
1.5 meters/second). Parastep training increased the size and strength of
quadriceps.
The
investigators stated that Parastep “is not used to increase ambulation
autonomy in daily life, but is used as an active means of exercise, in
order to prevent complications of immobilization, and to answer the
desire to stand and walk.” They concluded that “the psychological
benefits of the device are remarkable.”
2)
In a series of studies assessing different outcomes, Miami Project
investigators (USA) evaluated the Parastep training program. The
first examined the effects of training on walking ability, strength, and
various body measurements in 16 individuals (13 men, 3 women; average
age 29) with complete T4-T11 injuries sustained an average of 3.8 years
earlier. Subjects trained three times weekly for a total of 32 sessions.
The distance covered, the time spent standing and walking, and gait
speed steadily improved over the course of training, although there was
considerable performance variability between subjects. For example, six
subjects were able to ambulate more than 300 meters, but four could not
exceed 100 meters. Both thigh and calf girth increased, as well as the
amount of lean tissue (i.e., muscle).
In the
second study in this series, the investigators assessed the effect of
the 32-session, Parastep, ambulation program on overall body and
cardiovascular fitness. In other words, does this program have fitness
benefits above and beyond the improvements immediately associated with
walking? In this investigation, subjects were tested before and after
training by exercising with an arm ergometer, a device designed to
measure muscle power. Various fitness parameters were evaluated,
including the time it took to fatigue, peak workload, heart rate, upper
body strength, and various metabolic measures (e.g., oxygen uptake).
Training increased the time it took to fatigue, peak workload, and
oxygen uptake; and decreased heart rate. Upper extremity strength did
not significantly change.
The third
study in this series examined the effect of the Parastep training
program on bone density. The loss of bone density or osteoporosis is a
common consequence of SCI, aggravated, it is thought, by the individual
no longer being able to participate in weight-bearing activities. This
loss predisposes the SCI population to bone fractures. Researchers
measured bone density in several locations before and after subjects
completed the 32 Parastep training sessions. Although the training
program substantially increased weight-bearing activity, no increase in
bone density was observed.
The fourth
study looked at the exercise-related psychological effects that may
result from carrying out this 32-session Parastep program. These effects
were measured before and after training using a self-concept scale
designed to assess self worth and esteem, a depression measurement, and
individual subjective interviews. The results suggested that training
improved self concept and alleviated depression. In addition to the
psychological benefits often accruing from any intense exercise program,
the individual interviews suggested that participants were upbeat about
visible body changes, such as increase in girth and tone of quadriceps;
establishing a sense of connection with the lower half of the body; and
the restoration of a sense of normalcy by being able, for example, to
stand upright, even briefly, and interact with others face-to-face.
The final
study showed that the increased leg mass generated by the program was
associated with greater blood flow to the legs. This enhanced blood flow
was attributable to both exercise-catalyzed vascular structural changes,
such as increased blood-vessel diameter, and improved vascular control
mechanisms.
3) Dr.
Regine Brissot and
colleagues (France) evaluated the Parastep system in 15 individuals (11
men, 4 women) with T3-T11 thoracic injuries. All but two had complete
injuries, age ranged from 16-47 years, and the time since injury
averaged 53 months. Thirteen patients completed an average of 30
training sessions. Average walking distance without rest was 53 meters
(range 1-350 meters) with an average speed of 0.15 meters/second. As
with the previously discussed studies, all patients increased the
strength and girth of their quadriceps. One individual with an
incomplete injury was able to walk voluntarily without FES stimulation
after five training sessions. Psychosocially, patients noted a definite
improvement in self esteem and some progress in social integration.
Three years after training, five patients still used the device for
physical exercise but not for walking in a social setting.
The
investigators concluded that although “the Parastep approach has very
limited applications in daily life, because of its modest performance
associated with high metabolic cost and cardiovascular strain…it can be
a resource to keep physical and psychological fitness in patients with
spinal cord injury.”

4)
Manual Grasping Control:
Over the years, various FES devices have been
developed to enhance grasping in individuals with upper extremity
impairment. These devices also can be used as a rehabilitation tool to
improve voluntary manual control in some when used soon after injury.
Individuals with quadriplegia who use FES to
facilitate grasping report greater independence from adaptive equipment,
a reduced need for personal assistance, and improved self-image.
FES-grasping assistance can increase the number of activities an
individual can perform or improve existing abilities.
FES can facilitate both the lateral or key-pinch
grasp, effective for handling small objects, such as a spoon or a pen;
and the palmar grasp, used to hold a glass or a book.
Physical requirements for upper-extremity FES
include:
 | Hand and forearm muscles must be sufficiently
innervated. Too much denervation results in FES-initiated muscle
contractions that are too weak or fatigue too quickly for functional
use. |
 | Bicep, deltoid, and rotator cuff muscles must
have enough voluntary strength to control hand placement. |
 | Subjects must be able to see well enough to
direct their movements, especially if the hand lacks sensation. |
 | Truck support must provide a sufficient base
for controlled arm movements and object lifting. |
FES grasping devices can be subdivided in those 1)
that use surface stimulators that send current through the skin (i.e.,
transcutaneous), and 2) that require the implantation of stimulators
that deliver current directly to the targeted nerves.
Surface-stimulating devices include the
following:
1) The Handmaster
or Ness H200 is comprised of a hinged wrist-forearm splint with a
stimulator box electrically connected to the splint via a cable.
Electrodes
inside the splint deliver stimulus to key muscle points necessary for
movement. Ideally, candidates for this device should have sufficient
shoulder and biceps functioning with limited wrist extensors. One
potential disadvantage is that the device’s rigid splint design with
integrated electrodes may prevent the optimal placement of electrodes
for muscle stimulation.
Several studies have documented the benefits gained
from using the device in individuals with quadriplegia. For example, one
study evaluated the clinical experience of using it in 10 individuals
with cervical injuries ranging from the C4 to C6 level. These subjects
sustained their injuries 0.5 to 6 years before being recruited; their
age ranged from 21 to 65 years, and eight were men. In three subjects,
the splint could not be fitted due to, for example, size restrictions.
In six subjects, the device stimulated grasping and releasing, and four
subjects were able to do various grasping tasks (e.g., pouring water
from a can, putting a tape in a VCR, etc) that they could not do
otherwise.
Another study evaluated the use of the device in
seven individuals with C5 to C6 injuries sustained 3 to 17 years
earlier. In addition to various grasp and release assessments, outcome
measures included activities of daily living (ADL), such as picking up a
telephone, eating food with a fork, and lifting a videocassette. The
investigators reported significant improvements in hand function and
strength after subjects used the device for three weeks.
2) The Bionic Glove
is a fingerless flexible garment with a built-in stimulator and
electrode contacts. The device is controlled by wrist position to assist
in grasping and releasing. A pilot study in nine individuals with C6-7
injuries evaluated the effect of using the Bionic Glove for at least a
year as part of daily living activities. Grasping strength increased
four times, and the ability to carry out most manual tasks improved
substantially.
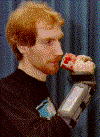
Another study
evaluated the benefits gained from six-months of using the Bionic Glove
in 12 individuals with C5-7 injuries. The investigators concluded that
the device increased both grasping power and range of movement. The
ability to carry out most manual tasks improved significantly. However,
individuals who already had some dexterity were more reluctant to use
the device.
3) The ETHZ-ParaCare, evolving into the
Compex Motion, device, is another flexible system that improves
grasping ability and strength in individuals with quadriplegia. The
rehabilitation benefit accruing by using the device relatively soon
after injury was the focus of a study with 11 subjects (age 15-70; 9 men
and 2 women) with C4-7 complete or incomplete injuries. All but two
sustained their injuries within eight months of initiating the program;
six started within three months. The investigators concluded that that
the technology can be used during early rehabilitation for different
purposes, including 1) for muscle training, 2) to support activities of
daily living, and 3) to facilitate the development of voluntary hand
movements. Some subjects discontinued using the device because the
device had increased voluntary grasping ability sufficiently so that
they no longer needed it. Others stopped because they required
assistance to place the electrodes at home or it took too long to put on
or take off.
4) The Belgrade Grasping System, evolving
into the ActiGrip System, initiates not only grasping but also
reaching through stimulating the triceps. This system allows flexibility
in electrode positioning to maximize muscle stimulation, but, as a
consequence, requires more time for the individual to place the
electrodes compared to the more rigid Handmaster device.
FES implantation grasping devices include
the following:
1) The Implantable Functional Neuromuscular
Stimulator or Freehand system probably was the most extensively
researched and evaluated FES-grasping device. It was implanted in 200+
individuals with C4-C5 injuries, obtained FDA approval, and was
commercially marketed by the NeuroControl Corporation. Unfortunately, in
spite of demonstrated effectiveness, it was withdrawn from the market in
2002 due to apparently economic reasons.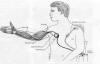
Basically, with this system, a joystick-like device
placed on the left shoulder is controlled through shoulder movement,
which, in turn, sends electrical signals to a nearby external
controller. This controller then sends signals to a transmitting coil
which is relayed to a receiver-stimulator that has been implanted near
the right shoulder. Finally, movement-generating impulse signals are
sent to eight electrodes implanted on the muscles of the right arm and
hand that are used for grasping.
To further augment hand function, various surgical
procedures are often carried out in conjunction with the implantation of
components, such as tendon transfers and joint fusions.
Because one must wait 18-24 months after injury
before all of these components can be surgically implanted, the Freehand
system can’t be used in efforts to regain voluntary hand movements in
the early stage of rehabilitation, as is the case with the
aforementioned surface-stimulation devices. Sometimes additional
surgeries are often needed to replace failed components and to
reposition stimulation electrodes.
The Freehand was evaluated in 51 subjects with C5-6
injuries recruited from eight SCI centers in the U.S., and one each in
the UK and Australia. Eighty-two percent of the subjects were men, time
sustained since injury averaged 4.6 years, and age averaged 32 years.
Subjects were followed at least three years. The device substantially
increased pinch force and grasp-release abilities in virtually all
patients. In addition, all subjects became more independent in carrying
out activities of daily living, such as eating with utensils, brushing
teeth, shaving, etc. Over 90% of the subjects used the device at home
and reported high satisfaction.
2) Myoelectrically-Controlled System: Based
on the Freehand system, a more powerful, less cumbersome,
second-generation device has been developed and studied in individuals
with SCI. Because the device eliminates the need to wear a shoulder
joystick as required with the Freehand, it is easier to put on and take
of.
With 12 stimulation electrodes, this system can
activate 12 muscles compared to only eight for the Freehand system,
allowing more refined hand function, forearm rotational movement, and
elbow extension. In addition to the stimulation electrodes, two
recording electrodes are implanted near muscles in which the
individual can still voluntarily control. Often, one recording electrode
is implanted on the muscle furthest down the arm that still has some
voluntary control, and the other is implanted in the neck or shoulder
region. In theory, however, even if the patient can voluntarily control
only one muscle, he still can use the device.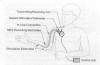
When the individual contracts the targeted
voluntary muscles, the recording electrodes pick up the electrical
signals (i.e., myoelectrical) the muscles generate and transmit them out
of the body to an external control unit. This unit transforms these
signals and relays them back into the body to the 12 electrodes that
stimulate the desired hand and arm function. In a nutshell, the
voluntary movement of controllable muscles send signals through the
device that stimulate paralysis-affected muscles.
In a typical procedure, like turning on a car
ignition with a key, the individual activates the system by moving the
shoulder or neck. Next, like shifting the car into gear to get it
moving, contracting and relaxing a forearm muscle with retained function
causes the hand to close and open, respectively.
One study looked at the functional gains accruing
by implanting the device into nine arms of seven individuals with C5-6
injuries sustained one to four years earlier (i.e., the device implanted
in both arms of two individuals). In all subjects, the device
substantially improved pinch force, grasp function, and ability to carry
out activities of daily living.
In a later study by the same investigators, three
individuals with C5-6 injuries using the device were followed for two to
four years. Augmentative surgeries, such as tendon transfers, were
carried out at the same time that the device was implanted. As before,
improvements were noted in pinch force, grasp function, and activities
of daily living. Several of the recording electrodes needed to be
surgical adjusted or repositioned.
3) The STIMuGRIP system is being developed
by Finetech Medical. With this technology, a receiver is implanted under
the skin of the forearm that relays signals to two electrodes connected
to grip-controlling muscles.
Like
a wristwatch, an external controller is strapped around the forearm
directly over the implanted receiver. The controller detects arm
acceleration somewhat similarly to a computer game sensing a forearm
swing to hit an imaginary tennis ball. This movement generates signals
which are sent to the internal receiver and, in turn, the electrodes
that trigger the stimulation or relaxation of various muscles used to
pick-up, hold, and release an item.

5)
Bladder/Bowel Management:
FES offers a potential bladder and bowel control mechanism for individuals
with SCI. Devices have included the Brindley (also called
Finetech-Brindley, Brindley Vocare) and the InterStim
systems. Both surgically implanted devices stimulate sacral nerves to
achieve desired effects.
The Interstim
was not specifically designed for SCI use. Unlike the Brindley device,
implantation of the InterStim does not involve the cutting of nerves.
Therefore the InterStim can be used to treat urinary incontinence in
individuals with complete and incomplete SCI.
Clinical
results using Interstim – not specifically for those with SCI – indicate
that reliable continence is achieved by 45%. An additional 34% of users
report that incontinence is reduced by 50% or greater.
The ideal Brindley candidate is an individual with
complete SCI who suffers incontinence and frequent urinary tract
infections. The system drains the bladder’s volume to less than 50
milliliters, which eliminates catheterization, reducing infections.
Brindley use is restricted to those with complete
SCI because it often requires the cutting of sacral sensory nerves and
bladder nerve roots, which block sensations needed for reflex erections
and precludes spontaneous improvements in voluntary bladder control.
Surgically implanted Brindley components include an
electrical stimulator, wire leads, and cuff electrodes. The stimulator
is implanted in the abdomen under the skin, usually beneath the ribs.
Silicon-coated electrodes are implanted around surgically exposed spinal
sacral roots. Implanted wire leads connect the components.
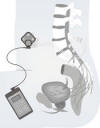
Using separate frequencies and pulse durations, an
external radio-frequency control device directs the Brindley system to
stimulate lower bowel contractions or reflex erections.
To use the Brindley system, patients must:
 | Be physically mature (skeletal growth after
implantation can dislodge implanted components); |
 | Possess a complete spinal-cord lesion; |
 | Be neurologically stable (in order to
manipulate the device and establish the right time to use it); |
 | Possess intact, peripheral nerves to bladder
and sphincter muscles, which can be stimulated; |
 | Have reflex bladder contractions, which
generate adequate bladder pressures. |
Many articles
have been published on the use of the Brindley device, including the
following summarized below:
Dr. Johannes Kutzenberger
et al (Germany) summarized 16
years of experience treating 464 patients with paraplegia (220 female,
244 male) with the Brindley device. Specifically, sensory nerve roots at
the sacral S2-5 level were completely transected – a procedure called
sacral deafferentiation. This selective cutting eliminates the sensory
input from key bladder muscles into the spinal cord, and, in turn stops
the reflex contraction of the bladder muscles, which may otherwise lead
to uncontrolled bladder emptying. The second step was the implantation
of the Brindley stimulator on the still intact motor nerve roots which
innervate the muscles needed for bladder control. Through the use of an
external transmitter, the stimulator allowed the patients to void
voluntarily. Hence, in a nutshell, bladder control was achieved by 1)
cutting the input nerves that trigger uncontrolled bladder emptying, and
2) establishing external control over the nerves that stimulate bladder
contraction.
Of the 464
treated patients, 440 have been continuously followed for 0.5 to 17
years. Continence was achieved in 83% of them. Frequency of voluntary
voiding averaged 4.7 times per day, and voluntary defecation averaged
4.9 times per week. In addition, urinary tract infections decreased from
an average of 6.3 per year before the procedure to 1.2 afterwards.
Dr. H.E. van der Aa
and colleagues (Netherlands)
reported the treatment of 38 patients with SCI with the Brindley system.
Of these patients, 33 were men, and age ranged from 15 to 59. All
patients had either thoracic or cervical injuries sustained at least a
year before treatment. Of the 38 treated patients, 37 were
retrospectively evaluated. All demonstrated an increase in bladder
capacity and a decrease in residual urine volume. Thirty-one were fully
continent. Patients also reported a decreased infection rate and
improved social life.

6)
Respiratory Support:
FES has provided respiratory assistance for individuals with higher-level,
respiration-compromising injuries. Although mechanical ventilation
provides respiratory support, it distorts the voice, limits mobility,
and increase infection risks. Using FES to stimulate diaphragmatic
contractions, called phrenic-nerve pacing, allows users to minimize
ventilator use. This can improve the subject’s mobility and speech,
while reducing respiratory secretions, respiratory infections, and
personal care needs.
Basically,
these pacing devices consist of surgically implanted receivers and
electrodes and an external transmitter and antenna. The transmitter and
antenna send a signal to receivers just under the skin. The receivers
transform the radio waves to puls es,
which then stimulate the phrenic nerves via electrodes. This nerve
stimulation triggers the diaphragm to contract and, as a result
inhalation. When the pulse stops, the diaphragm relaxes and exhalation
occurs. Appropriate pulsing will produce normal breathing (see
www.averylabs.com).
However, SCI between C3-5 can damage the
diaphragm-controlling phrenic nerves that FES stimulates. Therefore,
phrenic nerve functionality must be confirmed before phrenic-nerve
pacing is considered.
Dr. S. Hirschfield
and colleagues (Germany and Finland) compared the outcomes of treating
over a 20-year period 32 patients with functioning phrenic nerves with
pacing devices with 32 patients who were mechanically ventilated. The
mechanically ventilated patients were not randomized to this group but
rather could not use pacing devices because their phrenic nerves were
damaged. All patients had cervical C3-level or above injuries.
Although this
was not a controlled study due to the inherently different composition
of the two treatment groups, the investigators observed that treatment
of respiratory insufficiency after cervical SCI with a pacing device
instead of mechanical ventilation resulted in the following benefits:
·
Significantly reduces
upper airway infections,
·
Reduces cumulative
health-care cost,
·
Improves quality of
speech,
·
Improves quality of
life,
·
Reduces mortality and
prolongs life.
As demonstrated by Drs. Lloyd and Abbott Krieger
(USA), those with denervated phrenic nerves may be able to overcome this
obstacle through the surgical rerouting of one of the 11
rib-cage-associated intercostal nerves to the dysfunctional phrenic
nerve. At the same time, a phrenic nerve pacemaker is implanted. Of the
10 surgical nerve transfers, eight resulted in successful diaphragmatic
pacing. An average of nine months was required for transferred nerves to
innervate the diaphragms of these eight and respond to electrical
stimulation.
For individuals with one functioning phrenic nerve,
they may able to regain substantial respiratory capability by combining
the stimulation of inhalation-assisting intercostal muscles with
unilateral phrenic-nerve pacing. For example, Dr. Anthony DiMarco
et al (USA) did this combination procedure on for four individuals with
ventilator-dependent quadriplegia who still had a single functional
phrenic nerve. The intercostal muscles were activated by the electrical
stimulation of nerve roots through an electrode surgically placed in the
spinal cord’s thoracic area. After treatment, the subjects were able to
stay off mechanical ventilation for at least 16 hours per day. In
addition, improvements were noted in sense of smell, quality of speech,
and overall well being.
A less invasive procedure is
intramuscular-diaphragm pacing, a procedure which does not require the
cutting of phrenic nerves or the surgical opening of the chest (i.e.,
thoracotomy) usually required with conventional phrenic-nerve pacing.
Under this procedure, electrodes are laparoscopically placed in the
diaphragm near where the phrenic nerve connects to it. Because of the
method’s visualization capability, laparoscopic procedures only require
small incisions. After evaluating this approach in five
ventilator-dependent subjects with quadriplegia, Dr. Anthony DiMarco
and colleagues concluded that this technology provides comparable
ventilatory support and clinical benefit as conventional, much more
invasive, phrenic-nerve pacing.
FES-Assisted Cough: SCI-related abdominal
muscle impairment can affect coughing ability needed to clear airways of
secretions and irritants. In addition to clogging breathing airways,
coughing inability increases respiratory-infection risk and can lead to
atelectasis (a collapsed or airless state of the lungs). FES-assisted
cough is one mechanism by which coughing ability can be enhanced.
Basically, it involves taking a deep breath and then coordinating
FES-stimulated abdominal contractions with forced expiration.
In an illustrative case study, Dr. P.N. Taylor
et al (United Kingdom) evaluated the impact
of
electrical stimulation on blood-pressure control and cough augmentation
in a 40-year-old, ventilator-dependent male with a C3-4 level injury.
Before starting the stimulation program, the patient couldn’t cough on
his own and required manual assistance and tracheal suction to maintain
his airways. After initiating the program, he became independent in
coughing and no longer required suction or manual assistance.

7)
EPIDURAL ELECTRICAL STIMULATION In research reported in
2011, Dr. Susan Harkema and colleagues (USA) used epidural
electrical stimulation to improve functioning in a 23-year-old male with
a C7-T1 injury sustained 3.4 years before device implantation.
Although
possessing no motor function in trunk and leg muscles, he had retained
some below-injury sensation. Modified from an existing device used to
treat pain, an epidural spinal cord stimulation unit was placed over the
outer dura membrane of the patient’s spinal-cord L1-S1 segments. The
implantation intervention was combined with a rigorous rehabilitation
program involving body-weight-supported treadmill training.
The theory is that with a motor-complete cervical
injury of this nature, epidural stimulation can modulate “spinal cord
circuitry into a physiological state that enables sensory input, derived
from standing and stepping movements, to serve as a source of neural
control to perform these tasks.” In other words, the intact neural
networks remaining within the spinal-cord’s lumbosacral segments can be
reactivated with the right input. Basically, the implanted device will
generate signals that to some degree substituted for those normally sent
by the brain, and when these signals are combined with the sensory input
from the legs, uninjured neural networks can direct the movements
required to stand and step.
With epidural stimulation, the patient was able to
stand, supporting his full weight for periods ranging from 4-25 minutes,
feats he could not do before device implantation even with the extensive
locomotor training and rehabilitation he had undertaken. In addition,
the patient was eventually able to voluntarily move toe, ankle, and leg
muscles during epidural stimulation sessions. Finally, improvements were
noted in bladder, bowel, and sexual functioning, as well as temperature
regulation. The investigators concluded that “Task specific training
with epidural stimulation may have reactivated previously silent spared
neural circuits or promoted plasticity”
TOP
|