Before discussing specific transplantation
procedures, we need to briefly summarize the therapeutic potential of
various cells or tissues that are being incorporated into
cell-transplantation programs designed to restore some function after
SCI.
For readers desiring more in-depth information, the following books are
recommended: Stem Cell Now (Scott
CT. Pi Press; 2006), and Human Embryonic Stem Cells (Kiessling
AA, Anderson SC. Jones and Bartlett Publishers, 2007).
Olfactory Tissue/Cells
Because olfactory tissue is exposed to the air we
breathe, it contains cells with considerable turnover potential,
including renewable neurons, progenitor stem cells (below), and
olfactory ensheathing cells (OECs). Because of the unique nature of this
tissue, its regeneration-catalyzing potential is being examined for a
variety of neurological disorders, including in addition to SCI,
Parkinson’s disease and ALS (amyotrophic lateral sclerosis).
When transplanted into the injured spinal cord, OECs
potentially promote axonal regeneration by producing insulating myelin
sheaths around both growing and damaged axons, secreting growth factors,
and generating structural and matrix macromolecules that lay the tracks
for axonal elongation.
Stem Cells
Briefly, stem cells are precursor or progenitor cells
that have the potential to transform into a wide variety of tissue.
Although often dichotomously categorized as either embryonic or adult,
they actually represent a continuum of cell types that can transform
into our end-product tissue.
For example, as our central nervous system (CNS)
develops, embryonic stem cells generate more specialized tissue-specific
neural stem cells. In turn, these tissue-specific stem cells can
differentiate into neuron- or glial-restricted precursor cells, the
former with the potential to generate neurons and the latter into CNS
support cells called oligodendrocytes and astrocytes.
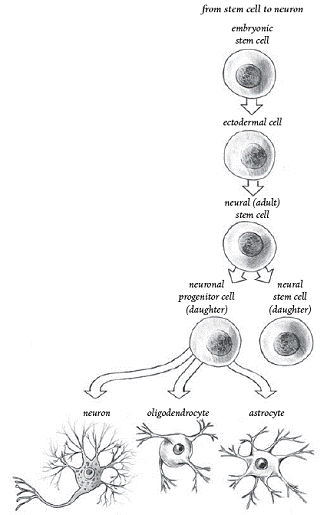
Stem-Cell Differentiation (From Stem Cell Now, CH Scott, 2005)
Omnipotent embryonic stem cells have the greatest
potential to differentiate into a wide range of cell types, although it
has been difficult to steer them in the desired direction. Adult stem
cells are found in most tissues, including, for example, CNS, bone
marrow, skin, intestine, liver, muscle, hair follicles, and even teeth.
Sometimes, they are robustly expressed, such as the bone-marrow’s
ongoing production of blood-cell-replenishing stem cells; in other
tissue, they are quiescent and need to be coaxed into action.
Although adult stem cells usually differentiate into
the specialized cells connected with the originating tissue, when
certain cues are provided, they can transform into cells associated with
other tissue. For example, under appropriate circumstances,
bone-marrow-derived stem cells can differentiate into nerve cells and,
indeed, are being used in several SCI-transplantation programs.
Furthermore, studies suggest that adult stem cells can reprogram back
into a more embryonic state. Finally, although we have emphasized their
therapeutic potential, given the wrong cues, stem cells can turn into
physiological troublemakers, causing, for example, cancer.
Embryonic Stem Cell Isolation
Basically, after an egg is fertilized, an embryo is
formed, which then splits into a two-cell embryo. In Stem Cell Now,
author Christopher Scott compares the process to dividing a soap bubble
with a knife, creating two smaller bubbles within the confines of the
original. Cut again, and it becomes four bubbles or a four-cell embryo.
This division goes on, successively creating 8, 16, 32, 64, 128-cell
embryo, the total volume changing little.
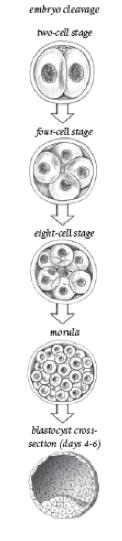
Embryo Cleavage (From Stem Cell Now, CH Scott, 2005)
Between four and six days, the cells rearrange into two layers: an outer
layer which will develop into placental and amniotic tissue and a few
dozen cells called the inner-cell mass (ICM) which turns into
everything else. Now labeled a blastocyst, the embryo is about 0.1-mm
across or the size of the period at the end of this sentence.
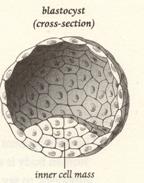
Blastocyst & Inner
Cell Mass (From Stem Cell Now, CH Scott, 2005)
As the cells continue to develop, they increasingly
lose their omnipotent nature. After about two weeks, the ICM cells start
to organize into three specific layers that become our various tissues:
1) ectodermal layer (developing into nerve, skin, etc), 2) mesodermal
(turning into blood, muscle, bone, etc), and 3) endodermal
(differentiating into the gut, liver, pancreas, bladder, etc.).
To obtain ESC, the ICM cells are isolated before
they start turning into these layers, and grown in culture. The
culturing technology has only recently emerged and requires
sophisticated methodology and skill. For example, scientists have had to
grow the cells on a layer of animal cells to provide nutrients and the
signals needed to keep the cells from further differentiating.
Schwann Cells
Schwann cells are responsible for remyelinating axons
in the peripheral nervous system, which, unlike the CNS, has
considerable inherent regenerative potential. Over the years there has
been much speculation on the potential of these cells to exert similar,
regenerative effects when introduced into the injured spinal cord.
Cell Source
Transplantable
cells can be obtained from the patient (autologous); genetically
different individuals, embryos, or umbilical cords (allogeneic); or
different species (xenogeneic). All three types have been transplanted
in an effort to restore function after SCI. Because autologous tissue is
from the patient, there is no immunological rejection. The
undifferentiated nature of embryonic and, to a lesser degree, umbilical
cells also minimizes rejection. Overall, cells are not selected based on
the theoretical best source or regenerative potential but their
isolation ease, such as concentrating blood stem cells.
Site of Transplantation
Donor cells are transplanted back into the patient
by a variety of routes, including into the spinal cord or surrounding
fluid, intravenously, or intramuscularly. Clearly, it’s easier and safer
to inject cells into a muscle, blood, or spinal fluid than surgically
accessing the spinal cord, albeit perhaps not as effective. Many
devil-is-in-the-details questions remain whether the cells transplanted
by these divergent routes actually reach the injury site to exert any
significant benefit. Although more studies are needed, investigators are
beginning to study the fate of transplanted cells in animal models of
SCI.
For example, Czech scientists are developing
procedures using magnetic resonance imaging (MRI). Basically, with
these procedures, very small magnetic iron-oxide particles are attached
to the stem cells, making them visible by MRI, and, in turn, allowing
them to be followed to some degree after transplantation. The overall
research goal is to determine the time course of migration to the injury
site and how long the cells persist there. With such information, we
can better understand the optimal time frame for transplantation, the
number of cells needed, and the best route of administration.
In another example, an international team of
scientists have used magnetic-imaging procedures to assess the migration
of iron-oxide-labeled olfactory ensheathing cells that have been
transplanted into the rat spinal cord. The labeled OECs
could be observed in the spinal cord for at least two months after
transplantation. Although extensive migration of transplanted OECs in
both directions was observed in the normal spinal cord, the cells
were unable to migrate through the injury-site scar of a transected
spinal cord.
The issue was further studied by Japanese
scientists in mice experimentally injured at the thoracic T10 level.
Mouse-derived neural stem cells, which were labeled with a
bioluminescent agent allowing later detection, were transplanted into
the injured animals by three different routes: 1) into the spinal-cord
injury site, 2) into the intrathecal space surrounding the cord, and 3)
intravenous administration. Six weeks later, the location and number of
surviving cells were assessed. When the cells were injected directly
into the injury site, 10% of them survived, including cells remaining at
the injury site. In the case of the intrathecally transplanted cells,
although some did, indeed, migrated to the injury site, only a miniscule
0.3 % cumulatively survived after six weeks. Finally, no intravenously
transplanted cells were detected at the injury site. However,
considerable luminescence showed up in the chest, suggesting pulmonary
embolism (a blockage of the lung’s pulmonary artery). One third of the
mice died immediately after the intravenous transplantation. The
investigators concluded that implanting the stem cells directly into the
injury site was the most effective and feasible transplantation method.
As a part of
related research described later, Drs. Fernando Callera and Claudio de
Melo (Brazil) examined the deposition of autologous (i.e., isolated from
the patient), magnetically labeled, bone-marrow-derived stem cells
transplanted into 10 patients. Age ranged from 21 to 45, and the
duration since injury varied from 2 to 13 years. Stem-cell-rich bone
marrow was aspirated from the iliac crest of the patient’s pelvic bone,
and the stem cells isolated and labeled with magnetic nanoparticles.
Five hours after aspiration, these magnetically labeled cells were
implanted into the patient’s spinal canal by lumbar puncture. In five of
the ten patients, 20-day post-transplantation MRI assessments (i.e.,
magnetic resonance imaging) indicated that the labeled stem cells had
migrated to the injury site but nowhere else in the central nervous
system. In a control group of six individuals with SCI who got an
injection of just the magnetic beads without stem cells, no lighted up
areas were observed at the injury site. The study demonstrates that at
least some of stem cells transplanted by this method truly migrate to
the injury site.
Age & Level of Injury
Other factors that may influence stem-cell
therapeutic effectiveness include age and level of injury. As shown by
UK researchers, this seems to be the case for bone-marrow stem cells,
which have been transplanted in a number of SCI-related programs. In
this study, bone marrow was isolated from iliac crest (i.e., an area of
the pelvis) of donors with SCI and grown in culture. Donor age ranged
from 23-66 years, and all had complete injuries sustained five months to
23 years before the bone-marrow tissue was collected. Although a limited
sample size, stem-cell growth in culture was greater when the cells were
isolated from younger patients and those with cervical injuries.
Dr. Carlos Lima, who
developed procedures for transplanting stem-cell containing olfactory
tissue into the injured cord, will not accept patients older than 40 due
to the diminution of regenerative potency in this tissue with age.
TOP